Microscopes have been our eyes into the invisible world, revealing intricate details of life and matter. From the tiniest microorganisms to the intricate structures of materials, microscopy has opened up a universe of knowledge that was once beyond our grasp.
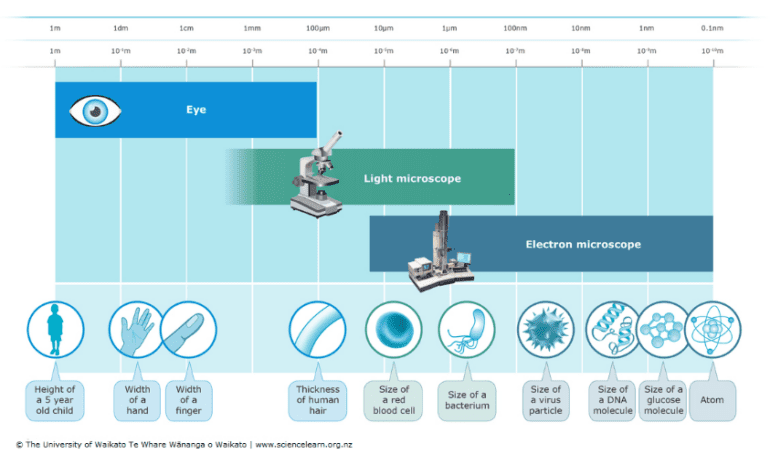
Figure 1: Resolving powers of the naked eye, light microscope, and electron microscope.
Source: Science Learning Hub
The journey into microscopy began in the late 16th century when Zacharias Janssen, a Dutch spectacle maker, is credited with creating the first compound microscope. This groundbreaking invention laid the foundation for the diverse array of techniques available today. These techniques vary widely based on the type of light used, the way images are formed, and the specific applications they serve.
In this exploration, we will delve into the fascinating world of microscopy techniques, uncovering their underlying principles, capabilities, and limitations. By understanding the strengths and weaknesses of different approaches, researchers can select the optimal technique to unlock the secrets hidden within their samples.
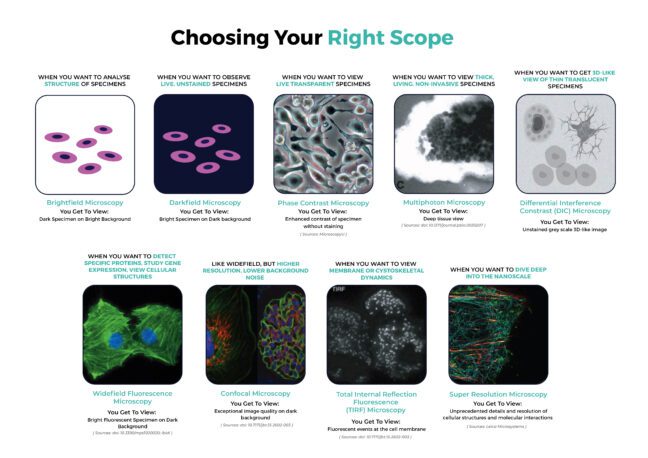
1. Brightfield Microscopy
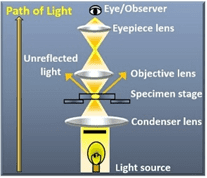
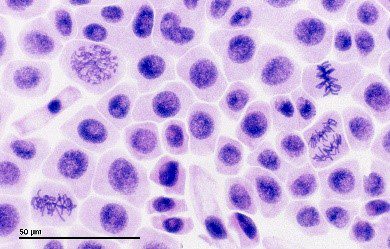
Figure 2: Brightfield Microscope optical set-up (Left). Brightfield image of onion cells in different phases of mitosis (Right).
Sources: Microscope Club; Wikimedia Commons
Brightfield microscopy is a fundamental technique in microscopy, widely used for its simplicity and reliability. As one of the most common methods in biology and medicine, it allows for the detailed observation of various samples.
How Brightfield Microscopy works?
Brightfield microscopy employs a basic optical system where light is gathered by the condenser, transmitted through the specimen and into the objective lens. The resulting image is formed due to variations in light intensity caused by the specimen’s absorption, reflection, and refraction of light. This contrast mechanism produces a dark sample against a bright background, hence the name. Brightfield microscopy is most effective on thin, translucent specimens that exhibit sufficient contrast. This contrast can be inherent to the sample, such as natural pigmentation, or artificially enhanced through staining techniques.
Applications of Brightfield Microscopy
The versatility of brightfield microscopy extends across numerous fields. Biologists and medical scientists utilise this technique to examine cells, tissues, and microorganisms. Materials scientists also employ brightfield microscopy to analyse the structure of materials.
Limitations of Brightfield Microscopy
While effective for samples with sufficient contrast, brightfield microscopy is challenged by transparent specimens. The technique’s ability to visualise details is limited by the contrast between the sample and its background.
2. Darkfield Microscopy
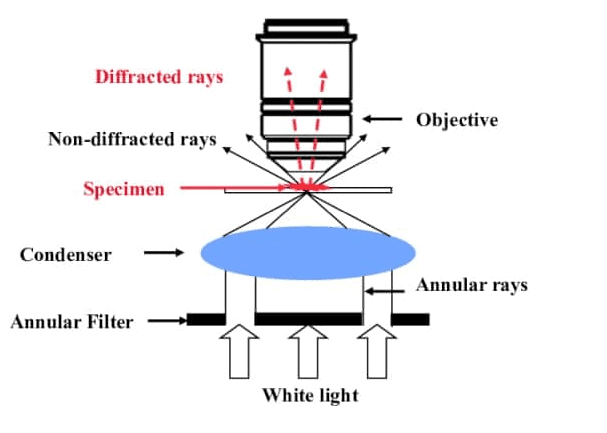
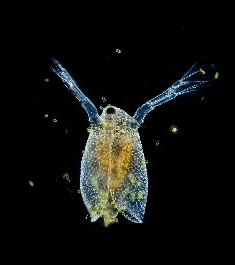
Figure 3: Darkfield Microscope optical set up (Left). Darkfield image of Ceriodaphnia dubia (Right).
Sources: Microscope Club; Wikimedia Commons
In contrast to brightfield microscopy, darkfield microscopy creates a striking image by blocking direct light from reaching the objective lens. Only light scattered by the specimen itself enters the lens, resulting in a bright sample against a dark background.
How Darkfield Microscopy works?
Darkfield microscopy achieves high contrast by blocking direct light from reaching the specimen. With a special condenser, light is directed at the sample from the sides, creating a hollow cone of illumination. When a specimen is present, it scatters the light, causing it to enter the objective lens. This produces a bright image of the specimen against a dark background, enhancing visibility, especially for unstained and transparent samples.
Application of Darkfield Microscopy
Darkfield microscopy is particularly useful for observing live, unstained specimens, such as bacteria, protozoa, and spirochetes. It is also employed in materials science to detect small particles and defects. It is effective for highlighting surface structures, imperfections, and boundaries in opaque samples.
Limitations of Darkfield Microscopy
The dark background can make it challenging to observe fine details within the specimen, while dust and debris can appear as bright objects, potentially obscuring the sample. In addition, the high light intensity required for darkfield can potentially damage sensitive specimens.
3. Phase Contrast Microscopy
Phase contrast microscopy is a powerful technique for visualising transparent specimens, such as living cells, that lack sufficient contrast for brightfield microscopy. It exploits the fact that different structures within a specimen have varying refractive indices, causing light to travel through them at different speeds.
How Phase Contrast Microscopy Works?
A special condenser and objective lens are used to convert phase differences (invisible to the human eye) into amplitude differences (visible as variations in brightness). This enhances the contrast of the specimen without the need for staining.
Applications of Phase Contrast Microscopy
Phase contrast microscopy is widely used in cell biology to study living cells, observe intracellular structures, and monitor dynamic processes. It is also employed in microbiology for examining bacteria and other microorganisms.
Limitations of Phase Contrast Microscopy
Phase contrast images often exhibit halo artifacts around the edges of structures, which can obscure details. The phase rings in the objective lens can slightly decrease the overall resolution compared to brightfield microscopy. It is also more costly due to the requirement of specialised phase contrast objectives and condensers than standard brightfield optics.
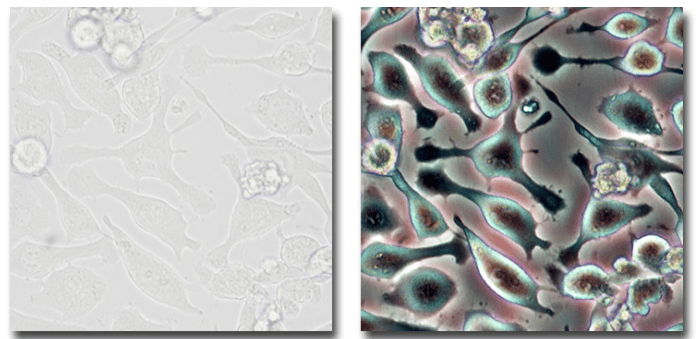
Figure 4: Images of human glial cells in Brightfield (left) and Phase Contrast (right).
Sources: MicroscopyU
4. Differential Interference Contrast (DIC) Microscopy
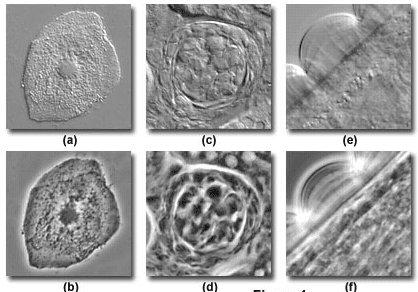
Figure 5: DIC (a, c, e) and Phase Contrast (b, d, f) images of a human buccal mucosa epithelial cell (a, b), a thick section of murine kidney tissue (c, d), and Obelia polypoid annulated stem perisarc
Sources: Olympus Lifesciences
Differential Interference Contrast (DIC) microscopy, also known as Nomarski interference contrast, is a sophisticated technique that produces striking, almost 3D-like images of transparent specimens. It excels at revealing subtle variations in refractive index within the sample.
How DIC Microscopy Works?
DIC employs polarised light and a series of optical elements to create two slightly offset beams of light that pass through the specimen. The differences in optical path length between these beams, caused by variations in the specimen’s refractive index, result in interference patterns that generate the image.
Applications of DIC Microscopy
DIC is particularly useful for studying unstained biological specimens, such as living cells, embryos, and microorganisms. The technique is also valuable in materials science for examining transparent or translucent materials.
Limitations of DIC Microscopy
DIC microscopy is best suited for thin, transparent samples with subtle refractive index variations. Its effectiveness diminishes significantly for thicker specimens, such as tissue sections, or those with strong pigmentation. As DIC requires specialised optical components, it increases the overall cost of the microscope system.
5. Widefield Fluorescence Microscopy
Widefield fluorescence microscopy is a fundamental technique that illuminates the entire sample with excitation light to visualise fluorescently labelled structures.
How Widefield Fluorescence Microscopy Works?
Fluorescence occurs when a fluorophore absorbs light at a specific wavelength (excitation) and emits light at a longer wavelength. To visualise target molecules, fluorophore-conjugated probes, such as antibodies or nucleic acid probes, are used to specifically label components of interest. The sample is then illuminated with excitation light, and emitted fluorescence is collected by the objective lens. A filter selectively transmits the emitted light while blocking the excitation light, producing an image where fluorescent structures appear bright against a dark background.
Applications of Widefield Fluorescence Microscopy
Widefield fluorescence microscopy is widely employed in biological research for immunofluorescence staining to detect and localise specific proteins. It is also used in in situ hybridisation to study gene expression patterns, and cellular imaging for visualising cellular structures and dynamics.
Limitations of Widefield Fluorescence Microscopy
While versatile, widefield fluorescence microscopy suffers from several limitations. Light emitted from regions above and below the focal plane contributes to background noise, reducing image clarity and contrast, especially in thick samples. Prolonged exposure to excitation light can damage photosensitive samples. Low fluorophore concentrations can result in weak fluorescence signals.
6. Confocal Microscopy
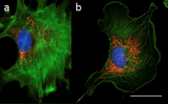
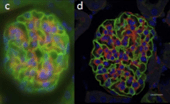
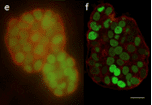
Figure 7: 3D images of widefield microscopy (a, c, and e)and confocal microscopy (b, d, and f) of epithelial cells labelled with DAPI (nuclear DNA-blue), Alexa 488-phalloidin (actin filament strain-green), and MitoTracker Red (mitochondria-red) (a, b), ∼20-μm-thick mouse kidney section, labelled with DAPI (nuclear DNA-blue), Alexa 488-wheat germ agglutinin (membrane stain-green), and Alexa 568-phalloidin (actin filament stain-red) (c, d), and 3D culture of MCF-10A mammary epithelial cell spheroid of ∼50 μm thick, labelled with a nuclear green fluorescent protein fusion and a red fluorescent protein fusion marking the membranes (e, f).
Sources: doi: 10.7171/jbt.15-2602-003
Confocal microscopy is a powerful technique that overcomes the limitations of widefield fluorescence microscopy by significantly enhancing image resolution and contrast.
How Confocal Microscopy Works?
Confocal microscopy uses a laser to scan the sample point by point. A pinhole aperture is placed in front of the detector, allowing only light emitted from the focal plane to be detected. This effectively eliminates out-of-focus light, resulting in sharper images.
Confocal microscopy employs various techniques to achieve high-resolution imaging:
- Laser Scanning Confocal Microscope (LSCM): This is the most common method, which utilises a focused laser beam to scan the sample point by point. While this approach offers exceptional image quality, it can be time-consuming, particularly for large samples or live imaging.
- Spinning Disk Confocal Microscope: This technique utilises a spinning disk with multiple pinholes to simultaneously illuminate different parts of the sample, accelerating image acquisition. As a result, it is often preferred for dynamic processes such as live cell imaging.
- Programmable Array Microscope (PAM): Similar to the spinning disk, it employs a patterned array of pinholes, but with greater flexibility in controlling the illumination pattern. However, this type of confocal microscope is less widely used compared to the other two.
Applications of Confocal Microscopy
Confocal microscopy is widely used in biological and biomedical research to study cellular structures, protein localisation, and dynamic processes. It is particularly valuable for imaging thick specimens, such as tissues and embryos, due to its ability to create optical sections.
Limitations of Confocal Microscopy
While confocal microscopy offers significant advantages over traditional widefield microscopy, it also has some limitations. Due to the point-scanning nature of confocal microscopy, acquiring images can be slow, especially for large datasets or high-resolution imaging. The intense laser light used in confocal microscopy can damage or bleach fluorescent samples, limiting observation time and affecting data quality. Confocal microscopes are expensive to purchase and maintain, requiring specialised equipment and trained personnel.
Explore Atlantis Bioscience’s Range of Cellular Stains including phalloidin, nuclear DNA, cell membrane, mitochondria, and many more!
7. Multiphoton / Two-Photon microscopy
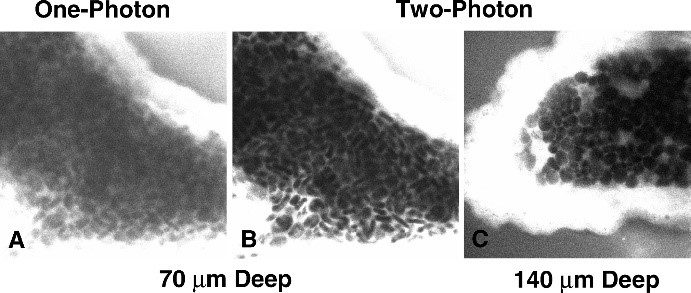
Figure 7: Comparison between Confocal Microscopy and Two-Photon Excitation Microscopy. (Right) Images of a Shark Choroid Plexus Stained with Fluorescein. The contrast of the confocal image (A) is significantly degraded at 70 µm, while two-photon excitation allows the collection of an image with excellent intensity contrast even at 140 µm. (Left)
Sources: doi: 10.1371/journal.pbio.0030207
Multiphoton microscopy or Two-Photon microscopy is an advanced imaging technique that goes beyond the capabilities of traditional confocal microscopy. It allows for deeper tissue penetration and reduced phototoxicity, making it an invaluable tool for studying living systems.
How Multiphoton Microscopy Works?
Instead of using a single photon to excite fluorophores, multiphoton microscopy employs two or more photons that simultaneously strike a fluorophore, causing it to emit a single photon of higher energy. Because the excitation light is in the infrared spectrum, it penetrates deeper into tissue compared to visible light used in confocal microscopy, enabling imaging of thicker samples. Due to the lower energy of the excitation light, the risk of photodamage to the sample is significantly reduced.
Applications of Multiphoton Microscopy
Multiphoton microscopy excels in imaging thick, living specimens due to its unique properties. It is suited for non-invasive studies imaging of brain structures, embryonic development, and organ physiology due to its ability to penetrate deeper into tissue. Because of its reduced phototoxicity, it enables live cell imaging and long-term observation of dynamic cellular processes without causing significant damage. It also offers the possibility for in vivo imaging to study biological processes in intact organisms, such as animal models of disease.
Limitations of Multiphoton Microscopy
The specialised equipment required, including high-powered femtosecond lasers and sensitive detectors, makes multiphoton microscopy a costly technique. While deeper than confocal microscopy, penetration depth is still constrained by light scattering and absorption within the tissue. Not all fluorophores are efficient for two-photon excitation, limiting the choice of labelling agents.
Looking for phalloidin stains for 2-photon imaging? Explore our phalloidin conjugates with dye options for super-resolution and 2-photon imaging
8. Total Internal Reflection Fluorescence (TIRF) Microscopy
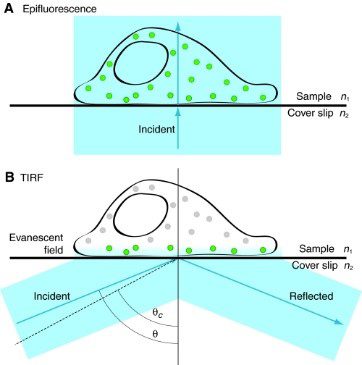
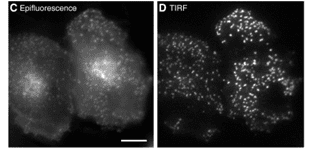
Figure 8: The physical basis of epifluorescence and TIRF illumination (A, B).
Comparison of images obtained using epifluorescence and TIRF (C, D)
Source doi: 10.1242/jcs.056218
TIRF microscopy is a specialised fluorescence technique that offers exceptional sensitivity and spatial resolution for imaging processes occurring near a cell surface.
How TIRF Microscopy Works?
When light undergoes total internal reflection at the interface between two media with different refractive indices (e.g., glass and water), an evanescent wave is generated. This wave penetrates a short distance (typically 100-200 nm) into the second medium. Only fluorophores within the evanescent field are excited, minimising background fluorescence from the bulk solution. This results in high signal-to-noise ratios.
Applications of TIRF Microscopy
TIRF is very useful in visualising membrane dynamics, such as receptor-ligand interactions, vesicle and protein trafficking, endocytosis, viral infection, cell-substrate interactions at the cell surface, or cytoskeletal dynamics.
Limitations of TIRF Microscopy
A drawback of TIRF microscopy is the necessity to use adherent cultured cells, limiting its use for suspension cells or certain types of tissues.
9. Super-Resolution Microscopy
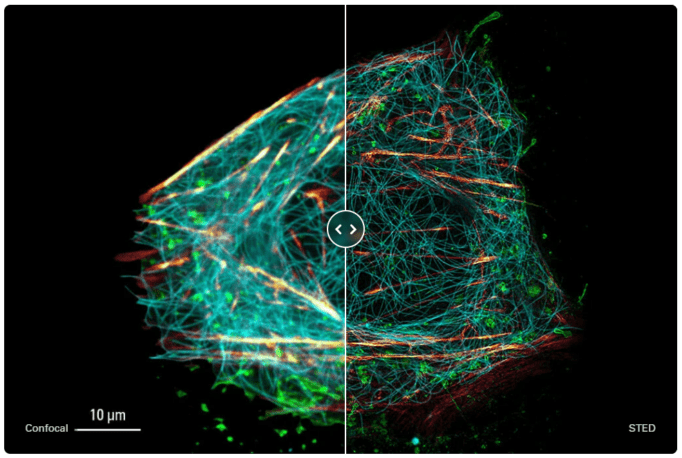
Figure 9: Live cell TauSTED image of U2OS cells, using labels for actin (SiR-actin, glow), microtubules (SPY555-tubulin, cyan), and membranes (CF488A coupled to WGA, green).
Source: Leica Microsystems
Super-resolution microscopy is a collection of techniques that surpassing the diffraction limit that traditionally constrains the resolution of light microscopes. This groundbreaking field has revolutionised our understanding of cellular and molecular processes.
How Super-Resolution Microscopy Works?
The diffraction limit, a physical constraint imposed by the wave nature of light, restricts the resolution of conventional light microscopy to approximately 200 nm. Super-resolution techniques employ various strategies to circumvent this limitation, enabling imaging at the nanoscale (5-20 nm). Generally, super-resolution techniques involve precise localisation of fluorophores within the sample, and computational reconstruction to combine information from multiple frames or measurements to create a high-resolution image. By manipulating the emission properties of fluorophores or using structured illumination, these techniques bypass the limitations imposed by the wavelength of light.
Several super-resolution techniques have been developed, each with its unique approach:
- Stimulated Emission Depletion (STED): Employs a second laser to restrict the emission of fluorescence to a small region, effectively increasing resolution.
- Structured Illumination Microscopy (SIM): Uses patterned illumination to generate interference patterns that enhance image details.
- Photoactivated Localization Microscopy (PALM) and Stochastic Optical Reconstruction Microscopy (STORM): Rely on the controlled switching on and off of individual fluorophores to precisely determine their positions.
How Super-Resolution Microscopy Works?
Super-resolution microscopy has found applications in a wide range of fields at nanoscale. These include the study of the organisation of cellular structures and dynamics of molecular interactions, imaging the fine structure of synapses and neuronal networks, characterising nanomaterials and their properties, and investigating the molecular basis of diseases.
Limitations of Super-Resolution Microscopy?
Super-resolution microscopy, while offering unprecedented detail, faces several challenges. As resolution increases, technical demands and limitations become more pronounced. These include the need for specialised fluorophores, susceptibility to environmental factors like vibration, and the potential for phototoxicity. Additionally, super-resolution systems are complex and expensive, often requiring significant investments in equipment and expertise. Balancing the desire for higher resolution with practical considerations, such as sample integrity and cost-effectiveness, is essential for successful super-resolution imaging.
Check out our validated CF® Dyes and cellular stains for Super-Resolution Microscopy.
References:
Bhakdi SC, Thaicharoen P. Easy Employment and Crosstalk-Free Detection of Seven Fluorophores in a Widefield Fluorescence Microscope. Methods Protoc. 2018;1(2):20. Published 2018 Jun 1. doi:10.3390/mps1020020
Jonkman J, Brown CM. Any Way You Slice It-A Comparison of Confocal Microscopy Techniques. J Biomol Tech. 2015 Jul;26(2):54-65. doi: 10.7171/jbt.15-2602-003. PMID: 25802490; PMCID: PMC4365987.
Mattheyses AL, Simon SM, Rappoport JZ. Imaging with total internal reflection fluorescence microscopy for the cell biologist. J Cell Sci. 2010;123(Pt 21):3621-3628. doi:10.1242/jcs.056218
Piston DW. When two is better than one: elements of intravital microscopy. PLoS Biol. 2005;3(6):e207. doi:10.1371/journal.pbio.0030207
https://www.sciencelearn.org.nz/images/526-resolving-power-of-microscopes
https://www.aps.org/apsnews/2004/03/lens-crafters-1590-invention-microscope
https://www.microscopeclub.com/bright-field-microscope
https://www.microscopeclub.com/dark-field-microscopy
https://www.microscopyu.com/techniques/phase-contrast/introduction-to-phase-contrast-microscopy
https://www.olympus-lifescience.com/en/microscope-resource/primer/techniques/dic/dicphasecomparison
https://ibidi.com/content/215-widefield-fluorescence
https://www.leica-microsystems.com/science-lab/super-resolution-microscopy-image-gallery