Gene therapy holds immense promise for managing or even curing diseases once considered untreatable. Conditions like certain cancers, β-thalassemia, and retinal dystrophy benefit from this approach, and the U.S. Food and Drug Administration (FDA) has already approved several gene therapy products for clinical use in these areas.
Gene therapy typically follows one of two strategies: ex vivo or in vivo transduction.
In ex vivo transduction, cells are collected from the patient or a healthy donor, genetically modified with vectors to alter their genotype, and then reintroduced as modified autologous or allogeneic cell products to replace deficient host cells. In contrast, in vivo transduction delivers the corrected gene in a carrier, like a virus, directly into the patient, enabling the vectors to interact directly with target cells to correct dysfunction.
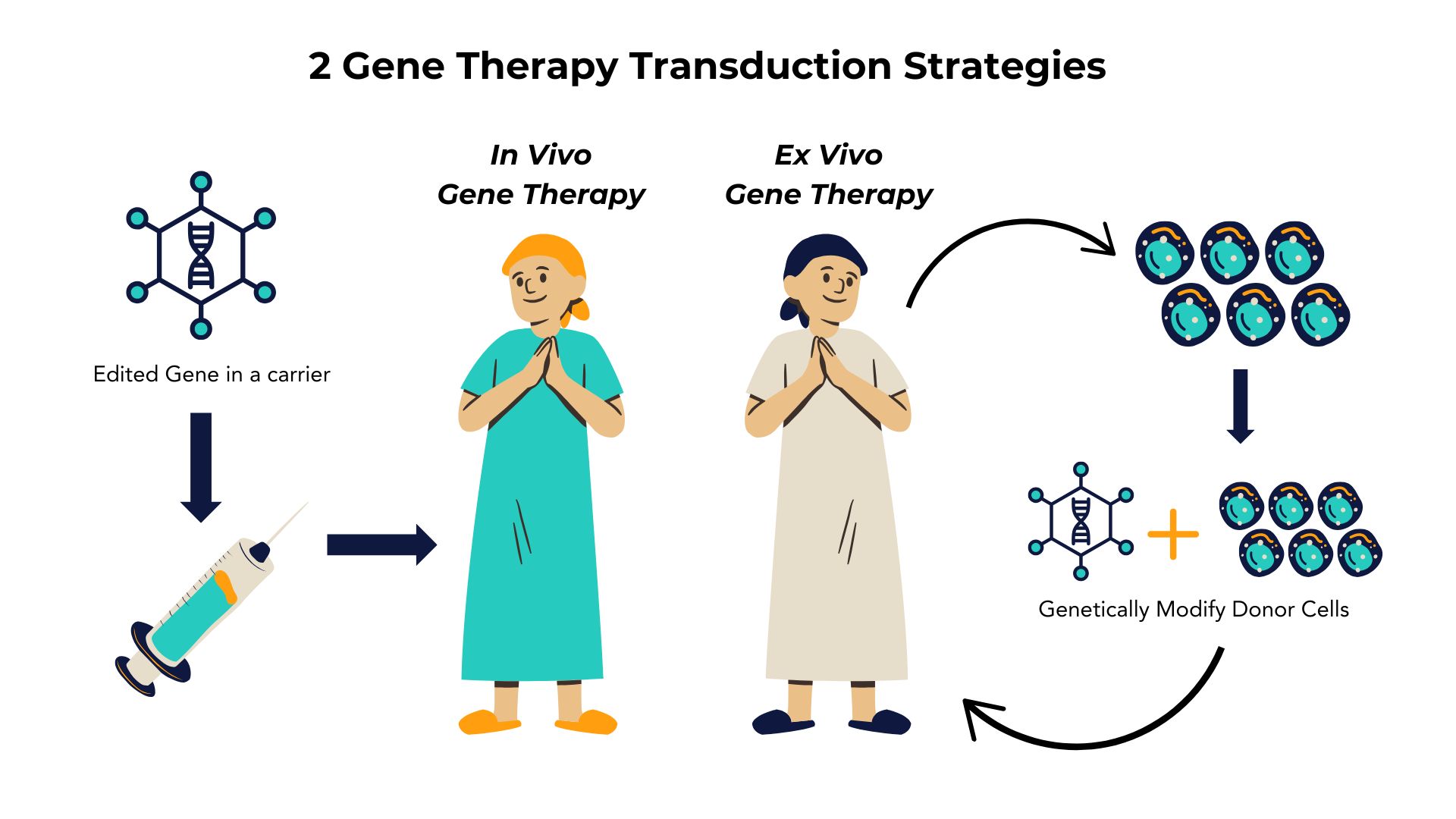
Viral vectors provide an efficient means for modifying target cells, and their use has become widespread in research and clinical applications. Several recombinant viral systems are available, and choosing the right one depends on factors such as your specific application, the size of your gene insert, and the target cell type.
Ƴ-Retroviruses
Gamma-retroviruses (Ƴ-retroviruses) are single-stranded RNA viruses that can integrate therapeutic genes directly into the host genome through reverse transcription, offering long-term gene expression, particularly in dividing cells. The most studied type of Ƴ-retrovirus is the Murine Leukemia virus (MLV). Key features of retroviral genomes include:
- Essential Genes
- Pol: Encodes reverse transcriptase, facilitating the conversion of RNA into DNA.
- Gag: Encodes core proteins for viral structure.
- Env: Encodes surface envelope proteins (Vesicular somatitis virus G glycoprotein; VSVG) necessary for target cell recognition.
- Ψ sequences: Enable viral packaging.
- LTRs: Found on both ends, these long terminal repeats aid the integration of DNA into the host genome.
Gene Therapy Mechanism of Ƴ-retroviruses
Retroviral vectors are generated by modifying viral genes to disable replication, ensuring only therapeutic genes are delivered. Typically, three plasmids are used:
- Plasmid 1 – Contains env but lacks Ψ, preventing virion formation.
- Plasmid 2 – Contains gag and pol without Ψ, supporting replication functions.
- Plasmid 3 – Contains the therapeutic gene, both LTRs, and Ψ sequences to enable virion assembly.
Advantages and Limitations of Ƴ-retroviruses
Retroviral vectors are widely used in hematopoietic stem cell (HSC) therapies due to their stable integration, which is effective for long-term expression. However, the random integration of retroviral vectors carries oncogenic risks, as they may unintentionally activate cellular oncogenes, increasing the risk of cancer. The packaging size is also limited to ~8 kb, restricting its application to smaller therapeutic genes, and retroviruses primarily target dividing cells, limiting effectiveness in non-dividing cells such as those in Duchenne muscular dystrophy (DMD) and cystic fibrosis (CF).
Strimvelis: A Retrovirus-Based Gene Therapy for ADA-SCID
Strimvelis is a retrovirus-based gene therapy approved by the European Medicines Agency (EMA) for the treatment of severe combined immunodeficiency (SCID) caused by adenosine deaminase (ADA) deficiency (ADA-SCID). In this therapy, a patient’s hematopoietic stem cells (HSCs) are collected ex vivo and transduced with a retroviral vector carrying a functional ADA gene. After transduction, the modified stem cells are reinfused into the patient’s body, enabling them to produce the missing ADA enzyme. This restoration of ADA production helps to restore immune function, offering a potential long-term solution for patients suffering from ADA-SCID. Strimvelis has been recognised for its ability to address the root cause of the disease and provide a durable cure for patients who previously faced limited treatment options.
Lentiviruses
Lentiviruses, derived from human immunodeficiency virus (HIV), are a subtype of retroviruses that share structural similarities with γ-retroviruses, such as genome architecture and host genome integration. However, unlike γ-retroviruses, lentiviruses can transduce both dividing and non-dividing cells, which significantly broadens their applications in gene therapy.
Lentiviral vectors utilise key genes—gag, pol, and env—for packaging, but they use slightly different isoforms from γ-retroviruses. Each lentivirus vector generation has improved safety through modifications to these packaging systems, optimising them for therapeutic use.
Evolution of Lentiviral Vectors
To enhance safety, lentiviral systems have evolved through several generations:
- First-generation vectors included accessory genes (vif, vpr, vpu, nef, tat, and rev) from the HIV genome, posing potential safety risks.
- Second-generation vectors removed several accessory genes (vif, vpr, vpu, and nef) that were not essential for gene transfer, increasing the safety profile without compromising transduction efficiency.
- Third-generation vectors split essential viral genes across three separate plasmids, remove the tat gene, and added self-inactivating (SIN) elements in the LTR to prevent unwanted gene activation in the host genome. This configuration is now widely used for clinical-grade lentiviral vectors, offering the highest safety level for therapeutic applications.
Advantages and Limitations of Lentiviruses
Lentiviral vectors allow stable, long-term expression of therapeutic genes by integrating into the host genome, even in non-dividing cells. This broadens their applications to various cell types and makes them effective for chronic genetic conditions. Lentiviral vectors also have low immunogenicity and multiple safety-enhanced generations, making them suitable for clinical use.
The integration into the genome, however, poses a risk of insertional mutagenesis, which can lead to cancer. Lentiviral vectors have a limited packaging capacity (~8-10 kb), restricting the size of the gene payload, and production is complex and costly. Additionally, although safer than earlier versions, immune response concerns still persist, especially with repeated dosing.
Gene Therapy Mechanism and FDA-Approved Lentiviral-Based Therapies
Lentiviral vectors have been optimised over the past two decades to improve their safety and versatility, especially for delivering therapeutic genes into HSCs. They have shown success in treating genetic disorders and cancers, with several approved therapies now in clinical use. Here are two examples of FDA-approved lentiviral-based therapies:
- Kymriah (tisagenlecleucel): This therapy is approved for treating acute lymphoblastic leukaemia (ALL) and large B-cell lymphoma. Patient T cells are collected and genetically modified using a lentiviral vector to express a chimeric antigen receptor (CAR) targeting the CD19 protein on cancerous B cells. The modified T cells are expanded and reinfused to target and destroy tumour cells.
- Zynteglo (betibeglogene autotemcel): Approved for beta-thalassemia, Zynteglo uses lentiviral vectors to deliver a functional beta-globin gene to the patient’s HSCs. After ex vivo transduction, the modified cells are reinfused, enabling the patient to produce functional haemoglobin, often achieving transfusion independence.
Adenoviruses
Adenoviruses, which initially gained attention for gene therapy due to their efficiency in transfecting cells, are known for causing mild respiratory infections in humans. Over 50 serotypes infect humans, and various non-human serotypes, like those from chimpanzees, provide a diverse range of options for vector design to suit specific therapeutic applications.
Adenoviruses possess a non-enveloped, double-stranded linear DNA genome, offering high maximum titers—a significant advantage for large-scale production and infection efficacy. With their ability to infect both dividing and non-dividing cells, adenoviruses do not require integration into the host genome, making them ideal for applications that demand high, transient gene expression or delivery of large genetic cargos (up to 36 kb in some vectors).
Types of Adenovirus Vectors
Adenovirus vectors are engineered to be replication-deficient by removing the E1 region, which controls replication, and substituting it with a therapeutic gene. Two primary types of vectors are commonly used:
- Standard Adenovirus Vectors: These vectors have a capacity of approximately 8 kb, making them suitable for moderate-sized gene inserts.
- High-Capacity “Gutless” Adenovirus Vectors: With most viral genes removed, these vectors can carry up to 36 kb of genetic material, allowing for stable expression and lower immunogenicity.
These vectors are usually produced in HEK293 cells, which express the E1 proteins needed for replication and packaging.
Advantages and Limitations of Adenovirus Vectors
- Advantages: Adenovirus vectors provide a large capacity for transgene insertion and can target both dividing and non-dividing cells across a wide variety of tissues. As the DNA remains episomal (non-integrated), adenovirus vectors are suitable for applications that require high but temporary gene expression, making them ideal for vaccine development and certain oncolytic (cancer-targeting) therapies.
- Limitations: One major drawback is the prevalence of pre-existing immunity, as many adults have been exposed to adenoviruses. This exposure can lead to a strong immune response that reduces the therapeutic efficacy of the vector. Strategies to mitigate this include using rare human serotypes, non-human serotypes (e.g., chimpanzee adenoviruses), polyethylene glycol (PEG) shielding, and encapsulation in alginate microspheres. However, immune responses and potential cellular toxicity still present challenges for broader applications.
FDA-Approved Adenovirus-Based Therapy
Adenovirus vectors have achieved FDA approval in therapeutic applications due to their versatility, particularly in cancer treatment. A key example is:
Adstiladrin (Nadofaragene Firadenovec): This adenovirus-based therapy is used for the treatment of high-risk non-muscle-invasive bladder cancer (NMIBC). Adstiladrin delivers a copy of the interferon alfa-2b gene directly into the bladder epithelium to stimulate a local immune response against cancerous cells. The gene is delivered via an adenovirus vector that allows for sustained production of interferon in the targeted area, providing a new therapeutic option for patients who may otherwise require more invasive treatments.
Adeno-associated Viruses (AAV)
Adeno-Associated Virus (AAV) vectors are derived from a non-pathogenic virus first identified over 50 years ago as a contaminant in adenovirus preparations. AAVs require co-infection with adenovirus for replication but do not cause disease on their own. Due to their low immunogenicity and favourable safety profile, AAV vectors are considered one of the most promising options for gene therapy. Read here to find out more about AAVs as a vector for Gene Therapy.
Structure and Serotypes of AAV
AAVs have a small, 5kb single-stranded DNA genome encased in an icosahedral capsid composed of three viral proteins (VP1, VP2, and VP3). Several naturally occurring AAV serotypes exist, each with distinct tissue tropisms, allowing for selective targeting of specific cells or organs in gene therapy. Capsid engineering can further optimise specificity, enhancing therapeutic potential by customising AAV variants with higher affinity for target tissues.
Advantages and Limitations of AAV Vectors
AAV vectors offer several advantages, including:
- Safety: AAVs are non-pathogenic and elicit a mild immune response, making them ideal for long-term gene therapy applications.
- Episomal DNA: Unlike retroviruses, AAV vectors do not integrate into the host genome, reducing the risk of insertional mutagenesis and oncogene activation. This episomal persistence provides stable expression of the therapeutic gene in target tissues.
- Targeted Delivery: With the ability to select specific AAV serotypes and design tissue-specific promoters, these vectors can be tailored to deliver genes precisely to the intended target tissue, such as the retina or liver.
Despite their advantages, AAV vectors face some limitations:
- Small Insert Capacity: The genome size of AAV is limited to approximately 4.7 kb, which restricts the size of the therapeutic genes that can be delivered. Techniques such as dual vector systems and minigenes are being explored to overcome this constraint.
- Production Challenges: Large-scale production of AAV vectors can be costly and challenging due to the need for significant amounts of high-quality virus. Additionally, a high proportion of empty capsids complicates vector purification processes.
- Genotoxicity Risks: Although AAV vectors do not integrate into the host genome, there is still a risk of genotoxicity if vector production involves contamination from packaging cell elements.
FDA-Approved AAV-Based Therapies
AAV vectors have shown great promise in clinical applications, with several FDA-approved therapies:
- Luxturna (voretigene neparvovec): An AAV-based gene therapy approved for the treatment of biallelic RPE65-mediated inherited retinal disease. Luxturna uses AAV2 to deliver a functional copy of the RPE65 gene directly to retinal cells, restoring vision in patients with this genetic disorder.
- Zolgensma (onasemnogene abeparvovec-xioi): An FDA-approved gene therapy for spinal muscular atrophy (SMA). Zolgensma uses an AAV9 vector to deliver a functional copy of the SMN1 gene to motor neurons, providing long-term therapeutic benefits for patients with SMA, a life-threatening genetic disorder.
Comparison of Common Viral Vectors Used for Gene Therapy
Ƴ-Retrovirus | Lentivirus | Adenovirus | AAV | |
Size | ~80-100 nm | ~80-100 nm | ~90-100 nm | ~25 nm |
Genome | ssRNA | ssRNA | dsDNA | ssDNA |
Packaging Capacity | ~8 kb | ~9 kb | ~8 kb – 36 kb | ~4.7 kb |
Transduction | Dividing cells | Dividing and non-dividing cells | Dividing and non-dividing cells | Dividing and non-dividing cells |
Transduction Efficiency | Moderate | Moderate | High | Moderate |
Key Characteristics | Integrates into the host genome, long-term expression | Integrates into the host genome, long-term expression | Non-integrating, High efficiency, broad tissue tropism | Non-integrating, episomal, Low immunogenicity, long-term expression |
Disadvantages | Potential insertional mutagenesis | Potential insertional mutagenesis | Transient expression, immune response | Small packaging capacity |
Expression | Stable | Stable | Transient | Transient or stable (depending on the application) |
Immunogenicity | Moderate-High | Moderate-High | High | Low |
Biosafety level | BSL-2 | BSL-2 | BSL-2 | BSL-1 |
Gene Therapy Strategy | Ex Vivo | Ex Vivo | In Vivo (can also be used ex vivo) | In Vivo (can also be used ex vivo) |
Common Application | SCID-X1, Adrenoleukodystrophy | Hemophilia, Cancer Immunotherapy, Neurodegenerative diseases | Cancer Therapy, Cystic Fibrosis, Inherited Blindness | Hemophilia, Inherited Blindness, Neurodegenerative Diseases |
Choosing The Right Vector for Your Gene Therapy Research
Selecting the optimal viral vector is a critical decision in gene therapy research. The choice of vector can significantly impact the efficacy, safety, and duration of gene expression. Several factors should be considered when making this decision:
Key Consideration:
- Target Tissue
- Dividing vs. Non-Dividing Cells: Lentiviruses are ideal for targeting non-dividing cells, while retroviruses are more suitable for dividing cells.
- Tissue Tropism: The natural tropism of a virus can influence its ability to infect specific tissues. For instance, AAVs have a broad tissue tropism, making them versatile for various applications.
- Desired Duration of Gene Expression:
- Long-Term Expression: Lentiviruses and AAVs can integrate into the host genome, leading to long-term gene expression.
- Transient Expression: Adenoviruses and some AAV serotypes can provide transient gene expression, which may be sufficient for certain therapeutic applications.
- Immune Response:
- Pre-existing Immunity: Pre-existing immunity to a specific viral vector can limit its efficacy.
- Immunogenicity: The immunogenicity of a vector can influence the duration of gene expression and potential side effects.
- Payload Capacity:
- Gene Size: The size of the gene to be delivered can influence the choice of vector. Lentiviruses and AAVs have relatively large payload capacities.
- Safety Profile:
- Insertional Mutagenesis: Retroviruses and lentiviruses can integrate into the host genome, potentially leading to insertional mutagenesis.
- Cytotoxicity: Some viral vectors, particularly adenoviruses at high doses, can induce cytotoxicity.
Practical Tips for Vector Selection:
- Consult with Experts: Seek advice from experienced researchers and clinicians to gain insights into vector selection and optimization.
- Consider Alternative Delivery Methods: While viral vectors are commonly used, other delivery methods, such as lipid nanoparticles and electroporation, may be suitable for specific applications.
- Optimize Vector Design: The design of the viral vector, including the choice of promoter and the sequence of the transgene, can significantly impact gene expression and safety.
- Conduct Preclinical Studies: Thorough preclinical studies, including animal models, are essential to assess the safety, efficacy, and biodistribution of the viral vector.
By carefully considering these factors, researchers can select the most appropriate viral vector for their gene therapy research and increase the likelihood of developing safe and effective treatments for genetic diseases. At Atlantis Bioscience we focus on building meaningful connections with and for the researchers we work with, to curate and develop translational solutions that help you bring your work from bench to bed efficiently. Connect or contact us to tap into our network of trusted experts from viral vector design and development to preclinical models.
References:
- Bulcha, J.T., Wang, Y., Ma, H. et al. Viral vector platforms within the gene therapy landscape. Sig Transduct Target Ther 6, 53 (2021). https://doi.org/10.1038/s41392-021-00487-6
- Leikas AJ, Ylä-Herttuala S, Hartikainen JEK. Adenoviral Gene Therapy Vectors in Clinical Use-Basic Aspects with a Special Reference to Replication-Competent Adenovirus Formation and Its Impact on Clinical Safety. Int J Mol Sci. 2023 Nov 20;24(22):16519. doi: 10.3390/ijms242216519.
- Milone, M.C., O’Doherty, U. Clinical use of lentiviral vectors. Leukemia 32, 1529–1541 (2018). doi.org/10.1038/s41375-018-0106-0
- Wang, JH., Gessler, D.J., Zhan, W. et al. Adeno-associated virus as a delivery vector for gene therapy of human diseases. Sig Transduct Target Ther 9, 78 (2024). https://doi.org/10.1038/s41392-024-01780-w
- Suzuki, Yasutsugu, and Youichi Suzuki. 2011. ‘Gene Regulatable Lentiviral Vector System’. Viral Gene Therapy. InTech. doi:10.5772/18155.
- Vargas, J.E., Chicaybam, L., Stein, R.T. et al. Retroviral vectors and transposons for stable gene therapy: advances, current challenges and perspectives. J Transl Med 14, 288 (2016). https://doi.org/10.1186/s12967-016-1047-x