Preclinical mouse models are essential in the drug discovery and development process for new cancer drugs. These mouse models come in different types, like syngeneic mouse models, genetically engineered mouse models (GEMM), cell line-derived xenografts (CDX), and patient-derived xenografts (PDX). Each cancer mouse model has its strengths and weaknesses, and the choice of the mouse model depends on what you are trying to achieve. Despite their differences, these mouse models have given us a wealth of information that helps us better grasp cancer pathobiology and predict how well treatments might work. In this blog, we’ll explore these mouse models and why they matter in the world of cancer research and drug development.
Syngeneic Mouse Models
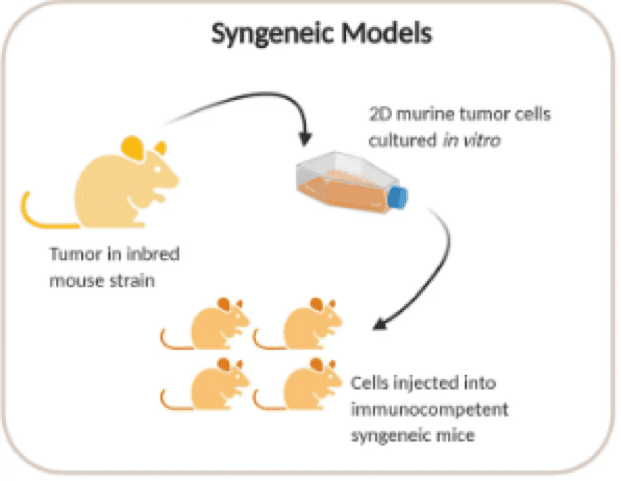
Credit: Bareham, B., Georgakopoulos, N., Matas-Céspedes, A. et al. doi.org/10.1007/s00262-021-02897-5. reproduced under the Creative Commons license
Syngeneic mouse models are among the earliest models used for oncology efficacy studies. These models involve transplanting allografts of immortalised mouse cancer cell lines into immunocompetent mice from the same inbred strain. Since syngeneic mouse tumours are immunologically compatible with their hosts, this minimises immune rejection and allows the tumours to grow.
Due to their intact murine immune system, syngeneic mouse models are particularly attractive for proof-of-concept immuno-oncology studies. They are cost-effective and easy to generate, as the cell lines can be readily cultured and expanded in any lab. Host mice are readily available, tumour engraftment is usually 100%, and studies can be conducted with statistically significant numbers of mice per group.
Various mouse cell lines have been developed for syngeneic models, primarily in the C57BL/6 and BALB/c backgrounds. These cell lines can be extensively characterised, including growth data, drug sensitivity, RNA sequencing, and other factors, allowing researchers to select the appropriate model based on their specific characteristics.
Syngeneic mouse models are increasingly used in combination studies, particularly in the investigation of checkpoint blockade or therapeutic interventions. Researchers can genetically engineer mice to express humanised immune checkpoint molecules (e.g., PD1, CTLA-4). Conversely, syngeneic cell lines can be engineered to express specific factors, such as checkpoint inhibitors, which have been instrumental in evaluating the efficacy of immune checkpoint drugs. Engineering syngeneic cell lines provides an expanded and humanised range of targets, to better investigate drug targets and explore biological mechanisms of action.
Genetically Engineered Mouse Models (GEMMs)
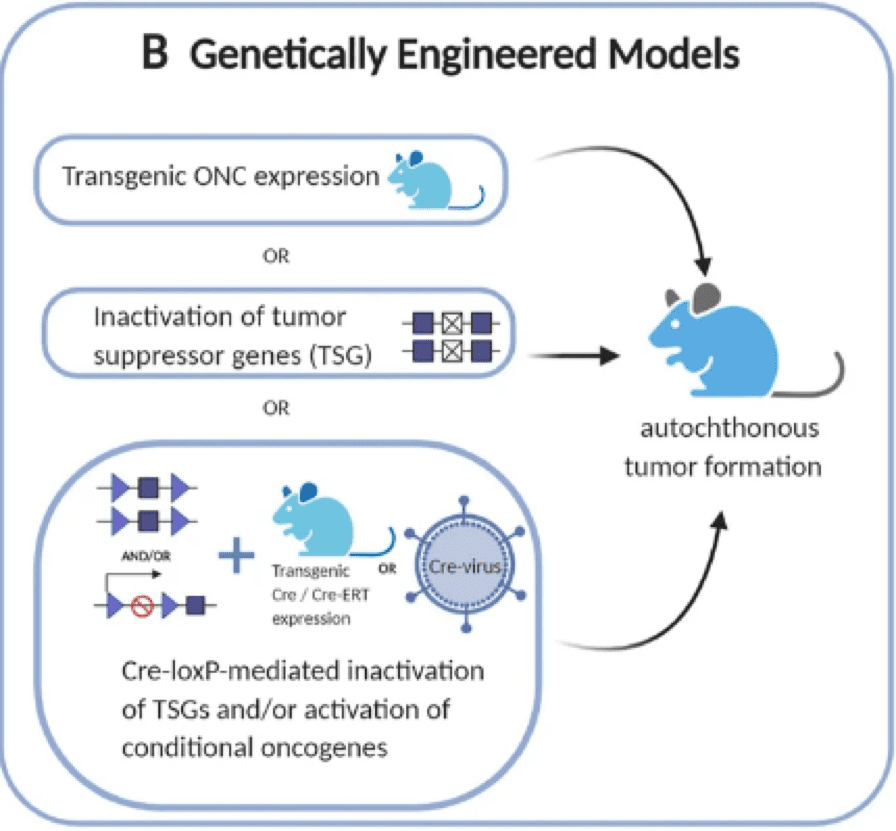
Credit: Bareham, B., Georgakopoulos, N., Matas-Céspedes, A. et al. doi.org/10.1007/s00262-021-02897-5. reproduced under the Creative Commons license
Genetically engineered mouse models (GEMMs) are immunocompetent mice engineered to develop specific types of cancer. These models are created by introducing genetic mutations or alterations known to be associated with human cancer into mice. GEMMs can accurately and robustly recapitulate primary cancers anatomically, histopathologically, and genetically, as they preserve the tumour microenvironment, including the immune, vascular, and stromal compartments. They also provide exquisite control over the genetics and kinetics of tumour initiation. Therefore, they play a critical role in the understanding of tumour initiation and progression.
Although GEMMs are capable of better predicting clinical responses, integrating them into the drug development workflow poses challenges. One limitation is the long experimental study duration, as it can take 2-10 months for tumours to reach the appropriate size before therapeutic intervention. Additionally, it is laborious and costly due to the production of colonies and the maintenance cost of genotyping and breeding. GEMMs may also exhibit unpredicted mutations (due to “off-target effects”) caused by the intrinsic properties of the methods used to develop them and may give rise to embryo-lethal phenotypes. Regardless of these challenges, recent advances in genome engineering with CRISPR/Cas9 technology have made GEMMs more accessible to researchers.
Cell Line-Derived Xenograft (CDX) Models
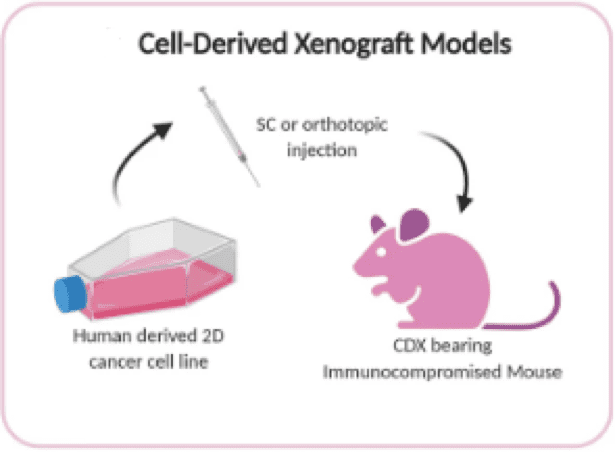
Credit: Bareham, B., Georgakopoulos, N., Matas-Céspedes, A. et al. doi.org/10.1007/s00262-021-02897-5. reproduced under the Creative Commons license
Cell line-derived xenograft (CDX) models are widely used in vivo models for anti-cancer therapies and are often employed for initial drug screening and the evaluation of anti-cancer agents. In CDX models, human cancer cell lines are injected into immunodeficient mice. These cells can be implanted subcutaneously, intravenously, or orthotopically to induce tumours in various microenvironments. Since the cell lines are of human origin, they must be transplanted into immunocompromised mouse models to prevent rejection. Some common immunocompromised mouse models include BALB/c nude mice, NOD/SCID, and NCG (triple-immunodeficient mouse model).
Similarly, to syngeneic mouse cell lines, human cancer cell lines can be extensively characterized. This allows for the selection of the appropriate cell line to establish the relevant cancer model. These cell lines are typically validated, enabling a smoother transition to in vivo studies after initial in vitro screening. However, it’s important to note that CDX models, while relatively straightforward for evaluating novel anti-cancer compounds, do not fully replicate the intra- and inter-tumour heterogeneity observed in human cancers due to the genetic homogeneity inherent to cell lines.
Patient-Derived Xenograft (PDX) Models
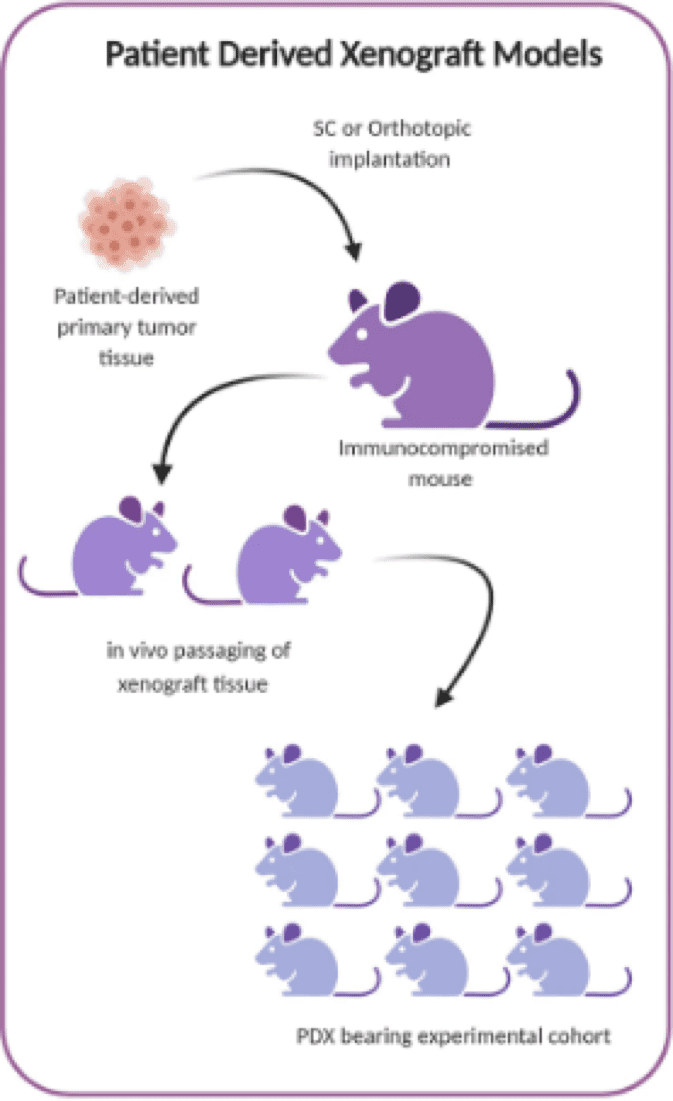
Credit: Bareham, B., Georgakopoulos, N., Matas-Céspedes, A. et al. doi.org/10.1007/s00262-021-02897-5. reproduced under the Creative Commons license
Patient-derived xenografts (PDX) models are the most translational preclinical model for cancer drug development. In this model, primary tumours from patients are implanted directly into immunodeficient mice, without prior propagation in cell cultures. PDX models closely mimic human disease because the implanted tumour tissue maintains the genetic and epigenetic abnormalities found in the patient, avoiding compromises from in vitro adaptation. Furthermore, they better reflect the heterogeneity and diversity observed in the human patient population.
To establish PDX models, primary tumours are cut into pieces and transplanted into immunocompromised mice, either subcutaneously or orthotopically. Once the tumours reach a size of 1–2 cm³, they are excised, cut into smaller sizes, and reimplanted to expand the tumour fragments. The first generation of PDX is called F1, and the subsequent generations are named F2, and F3. Typically, it takes an average of 2–4 months for the tumours to grow. Samples of the original tumour tissue and biopsies from different generations are usually preserved in freezers for subsequent genetic and proteomic evaluation. Generally, PDX models are established using low-passage patient-derived tumour tissue to preserve original tumour characteristics, including human stromal components. However, this also means that each model represents a limited resource.
Since PDX models lack an intact immune system, their use is limited primarily to chemotherapeutic drugs, as immunotherapeutic drugs necessitate an intact immune system. To address this limitation, humanised immune system mouse models were developed. These models involve reconstituting human immune cells (e.g., CD34+ hematopoietic stem cells or PBMC) into PDX models. To generate these mice, immunocompromised mice are first irradiated to enhance engraftment rates. Subsequently, CD34+ HSCs are intravenously injected into these mice, followed by engraftment of PDX tumours in mice with successfully engrafted human immune systems.
To ensure a high rate of engraftment, severely immunocompromised mice, such as the NCG (triple-immunodeficient mouse model), are the preferred choice. These mice have knockout Prkdc and Il2rg genes on the NOD background, making them excellent hosts for engrafting human tumours. Other variations of immunocompromised mice, such as NCG-SGM3 to support myeloid cell reconstitution and NCG-hIL15 for the reconstitution of functional T and NK cells, are valuable tools for reconstituting immune cells.
Comparison of Different Types of Preclinical Mouse Models
Syngeneic Tumour Mouse Model | Genetically Engineered Mouse Model | Cell-line derived Xenograft Model (CDX) | Patient-derived Xenograft (PDX) | |
Tumour Source | Immortalised mouse cancer cell line | Spontaneous tumour formation through genetically modification of oncogene or tumour suppressor gene | Immortalised human cancer cell line | Patient-derived tumours |
Immune competent/deficient | Immunocompetent | Immunocompetent | Immunodeficient | Immunodeficient |
Advantages | Reproducible and rapid growth Easy manipulated Subcutaneous and orthotopic | Preserves tumour microenvironment Tumour development driven by relevant alterations | Reproducible and rapid growth Easy manipulated Subcutaneous and orthotopic | Reproduces complexity of human disease |
Disadvantages | Lack of heterogeneity Does not fully replicate the complexity of the human immune system and tumour microenvironment | High maintenance and costly Breeding challenges Long experimental study | Lack of heterogeneity Lack of immunological agents Lack of tumour microenvironment | Generated in mouse with deficient immunity Low implantation rate |
Each of these mouse models has its advantages and limitations, and the choice of model depends on the specific research goals and questions being addressed. They have indeed generated an enormous amount of information that has advanced our understanding of cancer biology and drug development. Additionally, the use of multiple models in combination with other experimental approaches helps ensure more reliable and translational results in the quest to develop effective cancer therapies.
References:
- Bareham, B., Georgakopoulos, N., Matas-Céspedes, A. et al. Modeling human tumor-immune environments in vivo for the preclinical assessment of immunotherapies. Cancer Immunol Immunother 70, 2737–2750 (2021). https://doi.org/10.1007/s00262-021-02897-5
- Day CP, Merlino G, Van Dyke T. Preclinical mouse cancer models: a maze of opportunities and challenges. Cell. 2015 Sep 24;163(1):39-53. https://doi.org/10.1016/j.cell.2015.08.068
- Ireson, C.R., Alavijeh, M.S., Palmer, A.M. et al. The role of mouse tumour models in the discovery and development of anticancer drugs. Br J Cancer 121, 101–108 (2019). https://doi.org/10.1038/s41416-019-0495-5
- Kersten K, de Visser KE, van Miltenburg MH, Jonkers J. Genetically engineered mouse models in oncology research and cancer medicine. EMBO Mol Med. 2017 Feb;9(2):137-153. https://doi.org/10.15252/emmm.201606857
- Zeng Z, Wong CJ, Yang L, Ouardaoui N, Li D, Zhang W, Gu S, Zhang Y, Liu Y, Wang X, Fu J, Zhou L, Zhang B, Kim S, Yates KB, Brown M, Freeman GJ, Uppaluri R, Manguso R, Liu XS. TISMO: syngeneic mouse tumor database to model tumor immunity and immunotherapy response. Nucleic Acids Res. 2022 Jan 7;50(D1):D1391-D1397. https://doi.org/10.1093/nar/gkab804