So, what exactly are AAVs?
Adeno-associated viruses (AAVs) were first identified in 1965 by Bob Atchison and Wallace Rowe, who observed them as replication-defective particles within adenovirus samples. Belonging to the Dependoparvovirus genus within the Parvoviridae family, AAVs are unique in their dependence on a helper virus—such as adenovirus or herpes simplex virus—to complete their replication. In the absence of a helper virus, AAVs remain dormant in the host cell without causing disease, making them exceptionally safe for gene delivery applications.
In their wild-type form, AAVs can integrate into a specific site on human chromosome 19 (AAVS1), guided by the Rep protein. Recombinant AAVs (rAAVs), however, are engineered without Rep, thereby maintaining a stable, non-integrating presence within the cell. This episomal nature of rAAVs minimises the risk of insertional mutagenesis (mutation caused when exogenous DNA sequence integrates with the genome of host organism), enhancing their safety in gene therapy. AAVs are also extremely small, with a diameter of approximately 25 nanometers and a genome size of only 4.7 kilobases (kb), making them ideal for gene transfer into a variety of cell types.
With their safety profile, low immunogenicity, and flexibility for targeting specific tissues, AAVs have become leading tools in gene therapy and research, driving advancements across genetic and acquired disease treatments.
Key Characteristics of AAVs as Vectors
AAVs possess several unique characteristics and components that make them valuable vectors for research and therapeutic applications.
AAV Genome and Components
The AAV genome is a small, single-stranded DNA (ssDNA) molecule of about 4.7 kb, consisting of two essential open reading frames (ORF):
- The replication genes (Rep) ORF encoding four non-structural replication genes
- The capsid genes (Cap) ORF encoding three structural capsid genes.
Additional ORFs within the Cap gene, that encode for the membrane-associated accessory protein (MAAP), and assembly-activating protein (AAP) have been identified, although the functions remain unclear.
AAV Key characteristics include:
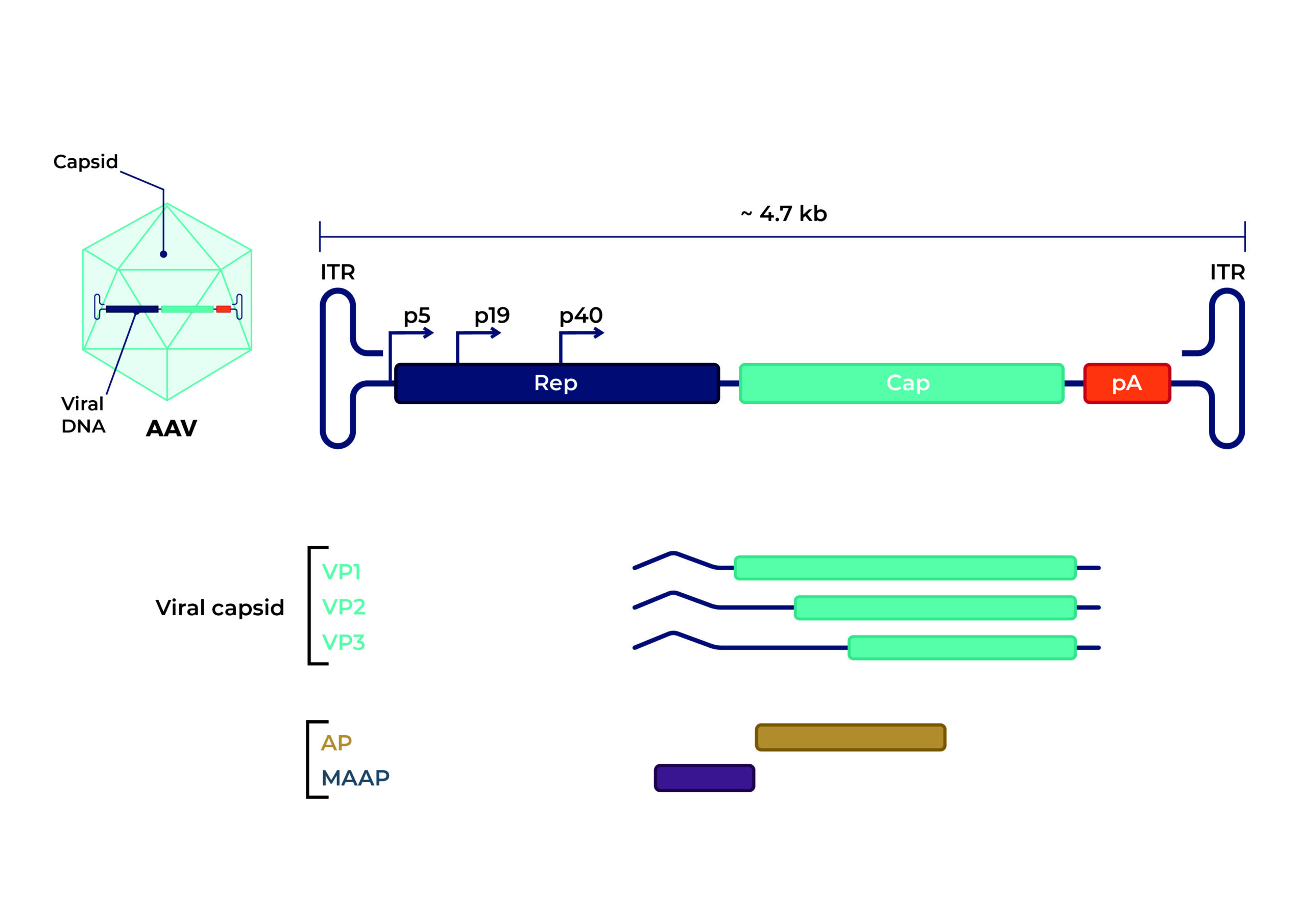
- Inverted Terminal Repeats (ITRs): The two 145-base ITRs located at each end of the AAV genome play a critical role in AAV replication. These repeats allow base pairing for complementary DNA strand synthesis.
- Rep Gene: The Rep gene encodes proteins essential to the AAV lifecycle, including Rep78, Rep68, Rep52, and Rep40, which are required for replication and packaging.
- Cap Gene: The Cap gene encodes the three capsid proteins (VP1, VP2, and VP3) that form the viral capsid at a ratio of 1:1:10, giving AAVs their ability to target specific tissues.
For gene therapy applications, a transgene of interest is inserted between the two ITRs within an AAV transfer plasmid. The Rep and Cap genes are supplied separately, in trans, during the production process.
AAV Helper Virus Requirement
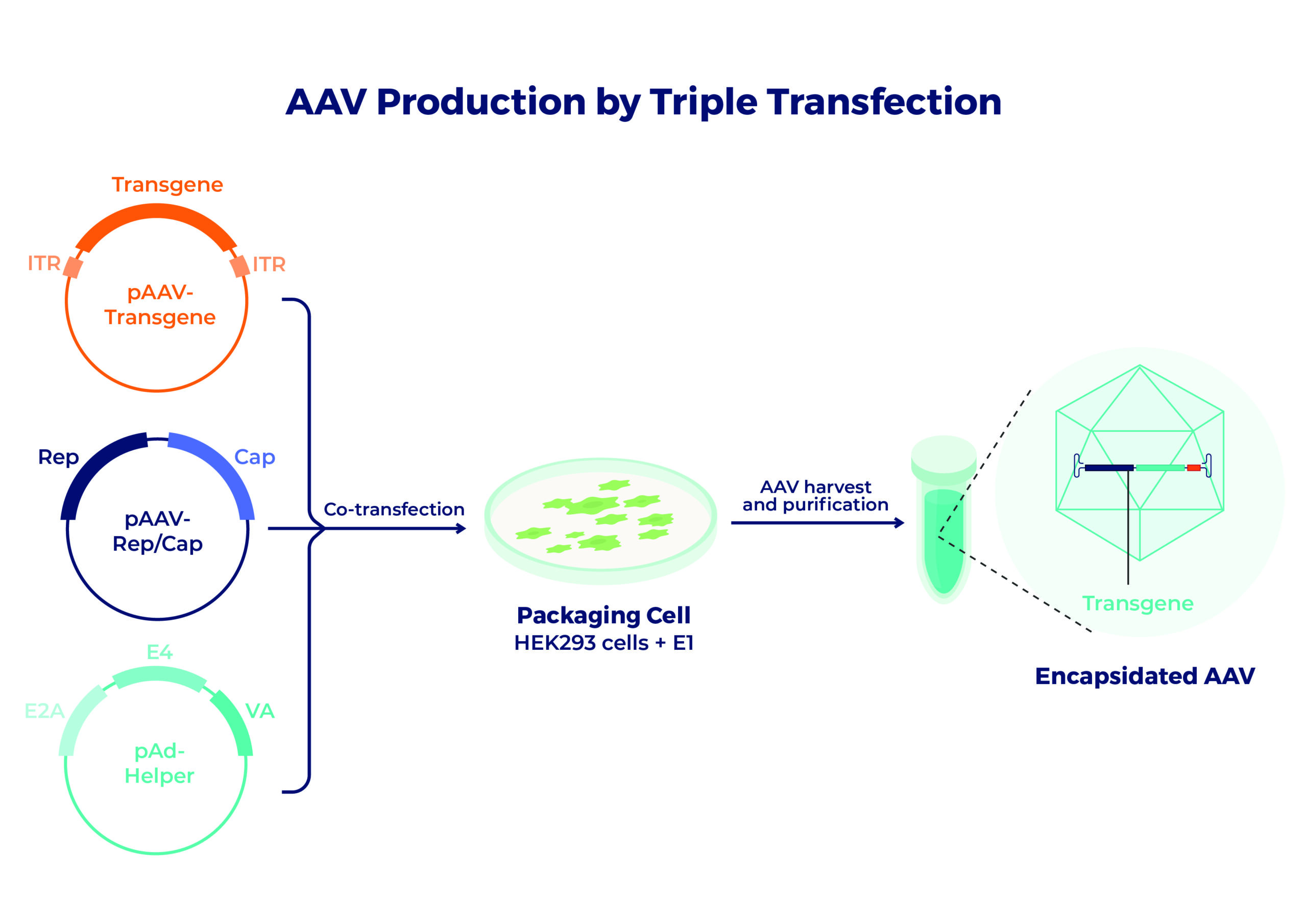
As a replication-incompetent virus, AAV needs a helper virus or helper plasmid for activation. Helper plasmids provide the necessary adenoviral genes—E4, E2a, and VA—that mediate AAV replication. In laboratory settings, the AAV transfer plasmid (containing the transgene), Rep/Cap plasmid, and a helper plasmid are co-transfected into HEK293 cells, which also supply the adenovirus E1 gene to produce infectious AAV particles.
AAV Serotypes and Tissue Tropism for Gene Therapy
AAVs exhibit significant diversity across their serotypes, each with unique tissue tropism—meaning they have natural preferences for specific cell types and tissues. This diversity enables scientists to select an appropriate AAV serotype to target particular tissues or organs, which is crucial in both gene therapy and research applications.
The natural capsid variations among AAV serotypes influence how well each serotype binds to certain cellular receptors, ultimately impacting which tissues are most effectively transduced. By choosing the appropriate serotype, researchers can enhance transgene delivery to targeted tissues while minimising off-target effects.
AAVs offer numerous advantages for gene therapy, including:
- Safety Profile: AAVs are non-pathogenic and elicit low immunogenicity, reducing the risk of adverse immune responses.
- Long-Term Gene Expression: They facilitate prolonged expression of therapeutic genes, beneficial for chronic conditions.
- Tissue Tropism: Their diverse serotypes allow for selective delivery to specific tissues, enhancing treatment efficacy.
- Minimal Insertional Mutagenesis: AAVs typically remain episomal, minimising the risk of oncogenic transformations.
Below is an overview of some of the most commonly used AAV serotypes and their preferred tissue targets:
AAV Serotype | Target Tissue | Description |
AAV1 | Skeletal muscle, heart | High transduction efficiency in muscle tissues |
AAV2 | Broad (liver, muscle, CNS, eye) | The most studied serotype, good for gene therapy research |
AAV5 | Lung, CNS | Preferred for targeting lungs and neurons |
AAV6 | Lung, muscle | Effective for lung and muscle gene transfer |
AAV8 | Liver, muscle | High liver tropism, often used in liver-targeted therapies |
AAV9 | Heart, CNS, liver | Able to cross the blood-brain barrier, useful in CNS applications |
AAV-DJ | Broad | Engineered for high transduction efficiency in multiple tissue types |
The choice of serotype is fundamental when designing AAV-based therapies or research studies, as it directly influences the efficacy and safety of gene delivery. For example:
- AAV8 and AAV9 are often chosen for liver and muscle applications due to their high transduction efficiency in these tissues.
- AAV9‘s ability to cross the blood-brain barrier has expanded its use in gene therapy for neurological disorders like spinal muscular atrophy (SMA) and certain types of muscular dystrophy.
- AAV2 is frequently used in ocular gene therapy, especially in retinal diseases, due to its excellent penetration of retinal layers.
By harnessing the unique tropism of each AAV serotype, scientists can design vectors tailored to target specific tissues, allowing for precise gene delivery and minimising potential side effects in non-target tissues. Additionally, capsid engineering and pseudotyping (swapping the capsid of one serotype with another) further refine these targeting capabilities, opening doors to a wider range of therapeutic and research applications.
Mechanism of Action of AAVs
The mechanism of action of AAVs involves several crucial steps, from entry into the host cell to the expression of the therapeutic gene. Understanding this process is essential for optimising AAVs for gene therapy applications. Here’s a detailed overview of how AAVs function:
- Entry into Host Cells: AAVs primarily enter host cells through receptor-mediated endocytosis, which allows them to bind to specific receptors on the cell surface. The interaction typically involves the viral capsid proteins on AAV binding to receptors such as heparan sulfate proteoglycans, AAV receptors (AAVR), and other cellular factors. This binding triggers the internalisation of the virus, leading to its entry into the cytoplasm.
- Endosomal Escape: Once inside the cell, AAVs are encapsulated in endosomes. To initiate the next phase, AAVs must escape the endosomal compartment. This escape can occur through pH-dependent changes in the capsid that lead to the exposure of endosomal membrane fusion peptides, which allows AAVs to release their genome into the cytoplasm.
- Transport to the Nucleus: After escaping the endosome, AAVs transport their ssDNA genome to the nucleus. This step is facilitated by the nuclear pore complexes, which allow small particles to pass through.
- Genome Conversion and Gene Expression: In the nucleus, the AAV genome undergoes conversion from a single-stranded form to a double-stranded DNA form, thanks to the host cell’s DNA repair mechanisms. This double-stranded DNA can then persist in the nucleus as an episome or, in the case of wild-type AAV, integrate into the host genome at the AAVS1 site on chromosome 19 via the action of the Rep proteins.
- For recombinant AAVs, which typically lack Rep, the double-stranded DNA remains episomal, allowing for stable expression of the transgene without integration. This episomal nature provides a level of protection from immune surveillance, allowing for sustained therapeutic effects.
- Transgene Expression: Once the AAV genome is converted and stabilised, the transgene inserted between ITRs can be transcribed and translated into the corresponding protein. The production of this protein depends on the regulatory elements incorporated within the transfer plasmid. For instance, the use of strong promoters can lead to high levels of transgene expression, while tissue-specific promoters can ensure targeted expression in specific cell types.
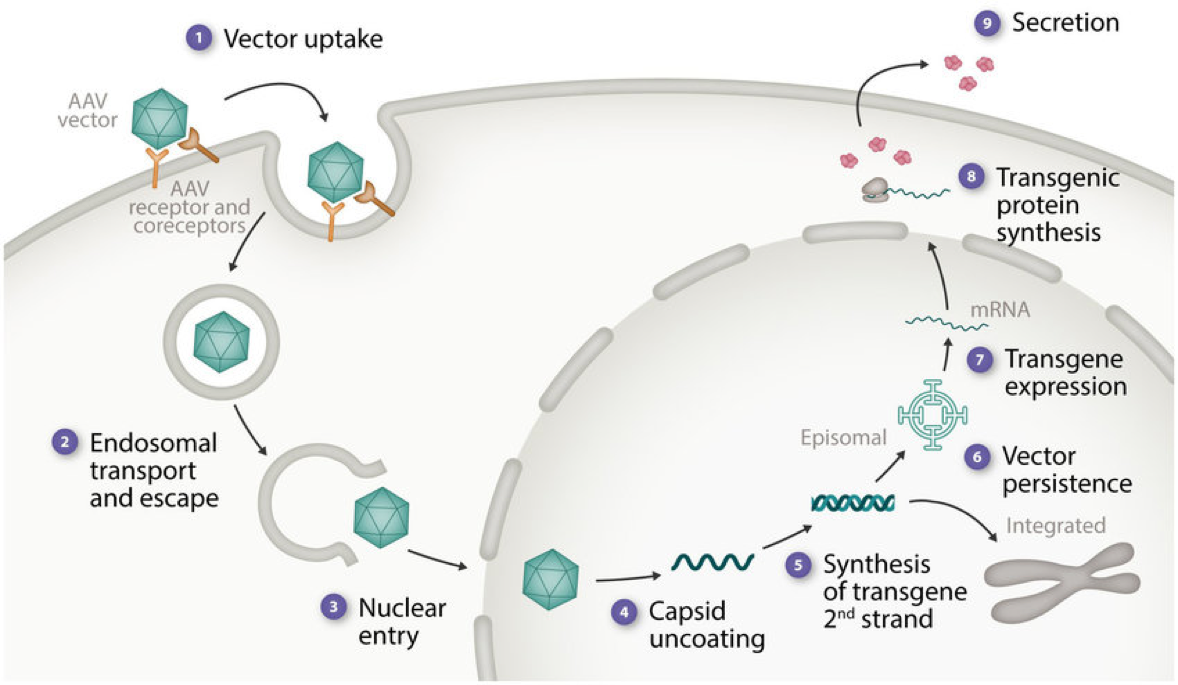
Credit: Batty, P and Lillicrap, D DOI: 10.1097/HS9.0000000000000540
Reproduced under the Creative Commons license HemaSphere
AAV vectors for Gene Therapy
Gene therapy modifies gene expression or alters cellular and tissue functions to treat disease, encompassing techniques like gene replacement, inactivation, or introduction of new genes. AAVs have emerged as one of the most effective vectors for gene therapy, offering promising solutions across a range of genetic and acquired diseases. Their unique properties—such as low pathogenicity, stability, and targeted tissue tropism—make AAVs particularly suitable for delivering therapeutic genes to specific cells. This potential has led to significant breakthroughs in treating previously intractable conditions, with AAV-based therapies becoming some of the first gene therapies to receive FDA approval.
Here’s an overview of key FDA-approved AAV-based gene therapies and the types of diseases suitable for AAV therapy.
FDA-approved AAV-based Gene Therapy
AAV-based gene therapies have demonstrated remarkable success in clinical settings, leading to multiple FDA approvals for therapies targeting rare and life-threatening diseases:
- Leber Congenital Amaurosis (LCA): Luxturna (voretigene neparvovec-rzyl), one of the first FDA-approved AAV-based gene therapies, treats LCA by delivering a functional copy of the RPE65 gene. LCA is a rare genetic disorder that causes severe vision impairment or blindness from birth. Clinical trials have shown significant improvement in patients’ vision, highlighting AAV’s transformative potential for treating genetic blindness.
- Spinal Muscular Atrophy (SMA): Zolgensma (onasemnogene abeparvovec-xioi) uses AAV9 to deliver a functional copy of the SMN1 gene to patients with SMA, a severe genetic condition affecting motor neurons that can lead to muscle weakness and early mortality. Zolgensma has demonstrated significant improvements in motor function and extended survival in infants diagnosed with SMA.
- Hemophilia: Hemophilia gene therapies leverage AAV vectors to boost clotting factor production, with several AAV-based treatments achieving promising outcomes. Hemgenix and Beqvez use AAV vectors to deliver Factor IX to hemophilia B patients, while Roctavian, an AAV5-based therapy, has shown sustained factor VIII expression for hemophilia A patients. These therapies offer a major advancement for hemophilia management, reducing the need for frequent clotting factor injections.
- Duchenne muscular dystrophy (DMD): Elevidys (delandistrogene moxeparvovec-rokl) addresses DMD, a severe genetic disorder that causes progressive muscle weakness and deterioration. By delivering a modified version of the dystrophin gene (micro-dystrophin), Elevidys helps restore some muscle function, compensating for the lack of functional dystrophin and providing new hope for patients with DMD.
Types of Diseases Suitable for AAV Therapy
AAV therapies are being developed for a variety of disease indications, particularly those that are neurologic, ophthalmologic, metabolic, neuromuscular, and cardiovascular. Each of these categories presents unique opportunities for targeted gene therapy.
- Neurologic Diseases: AAVs are being explored for conditions such as Parkinson’s disease and Huntington’s disease, which involve neurodegeneration. The ability of AAVs to deliver therapeutic genes directly to the central nervous system allows for sustained expression, potentially altering disease progression and improving patient outcomes.
- Ophthalmologic Diseases: AAV therapies have shown promise in treating inherited retinal diseases, such as Leber’s congenital amaurosis and retinitis pigmentosa. These conditions often stem from specific genetic mutations, making them ideal candidates for gene replacement therapies that aim to restore vision.
- Metabolic Diseases: AAVs are being investigated for their potential to treat metabolic disorders, such as phenylketonuria (PKU) and Wilson’s disease. By delivering genes that correct metabolic pathways, these therapies could help manage or even reverse the effects of these genetic conditions.
- Neuromuscular Diseases: Conditions like SMA and DMD are key targets for AAV gene therapies. These diseases are often caused by mutations in genes critical for muscle function, and AAVs can facilitate the delivery of therapeutic genes that promote muscle health and function.
- Cardiovascular Diseases: AAVs are also being explored in the context of heart disease, where they can be used to deliver genes that enhance cardiac function or protect against detrimental processes. This approach has the potential to address a range of cardiovascular conditions, from ischemic heart disease to heart failure.
The Shift to More Common Diseases:
While initial AAV therapies have focused on rare conditions, there is a growing interest in extending these approaches to more common diseases. As research progresses, the goal is to leverage AAV technology for a wider array of conditions, addressing significant unmet medical needs in larger patient populations. This shift is driven by advancements in gene therapy and an increasing understanding of the potential for AAVs to provide durable treatments for both rare and prevalent diseases.
Challenges and Limitations of AAVs
AAVs have significant potential for gene therapy but are not without limitations that researchers and clinicians must address:
- Pre-existing Immunity: Many people have pre-existing neutralising antibodies against common AAV serotypes, resulting from previous exposure to wild-type AAV. This can reduce the efficacy of AAV-mediated gene delivery, particularly in clinical settings. To address this, researchers use patient screening and serotype selection strategies to minimise immune interference.
- Limited Transgene Capacity: AAV vectors have a maximum packaging capacity of approximately 4.7 kb, which restricts the size of therapeutic genes or payloads they can carry. Larger therapeutic genes or complex gene constructs may require dual AAV systems or alternative approaches to increase delivery efficiency.
- Variability in Transduction Efficiency: AAV transduction efficiency is not uniform across all tissues and cell types, influenced by serotype selection, administration route, and the presence of specific cellular receptors. Researchers must carefully optimise these variables for each application to achieve effective and targeted gene delivery.
- Episomal Stability: While AAV DNA remains largely episomal, reducing the risk of insertional mutagenesis, this episomal form may be less stable in dividing cells, potentially affecting long-term therapeutic gene expression.
- Potential Genotoxicity: Although AAVs generally exhibit a favourable safety profile, there remains a risk of insertional mutagenesis if AAV DNA integrates into the host genome. This integration could lead to oncogenic transformations, necessitating thorough safety evaluations, particularly for long-term therapies.
- Manufacturing and Quality Control: One major hurdle for advancing AAV gene therapies lies in manufacturing and quality control. Current production methods often require large, specialised facilities, high labour costs, and extensive development timelines to meet clinical-grade GMP standards. Traditional methods developed in academic labs are difficult to scale, and batch-to-batch variability further complicates production, potentially affecting both safety and efficacy. Ensuring a high ratio of full, functional capsids is also challenging, as inconsistent ratios may dilute the therapeutic dose delivered. Additionally, capsids sometimes package unintended or non-therapeutic DNA sequences, raising safety concerns. Capacity constraints, a shortage of trained experts, and strict regulatory standards further add to the complexity and cost of producing consistent, high-quality AAV products.
AAVs in Research Settings
AAVs are not only instrumental in gene therapy but also serve as valuable tools in various research applications. Their ability to deliver genetic material with precision and efficiency makes them essential for genetic engineering, transgenic expression, and creating knockout models. Additionally, the integration of CRISPR technology with AAVs is revolutionising the field of genetic research.
Genetic Engineering and Transgenic Expression
AAVs are widely employed in genetic engineering to facilitate the expression of transgenes in both in vitro and in vivo settings. Researchers utilise AAV vectors to introduce new genes into organisms, allowing for the study of gene function, protein interactions, and the impact of genetic modifications on phenotypes. The benefits of using AAVs for transgenic expression include:
- High Efficiency: AAVs can transduce a wide variety of cell types, leading to high levels of transgene expression.
- Long-Term Expression: Once delivered, the transgenes can remain episomal in the host cell nucleus, allowing for sustained expression without the risks associated with random integration.
- Minimal Immunogenicity: AAVs are generally well-tolerated in host organisms, reducing the likelihood of immune responses that could compromise research outcomes.
This capability has facilitated the generation of animal models that mimic human diseases, providing critical insights into disease mechanisms and potential therapeutic interventions.
AAVs in Knockout Models and CRISPR Applications
AAVs play a pivotal role in creating knockout models to study gene function by delivering tools like CRISPR/Cas9 to specific gene targets. Knockout models allow researchers to inactivate genes, gaining insights into gene function, genetic interactions, and potential therapeutic targets.
By combining AAVs with CRISPR technology, researchers can deliver Cas9 and guide RNA (gRNA) to induce double-strand breaks at target genes. The errors introduced during the repair process effectively knock out the gene of interest, allowing researchers to investigate its function and impact.
The synergy between AAVs and CRISPR has opened new frontiers in genetic research by enabling targeted, efficient gene editing in living organisms. This combination provides several advantages:
- Targeted Gene Editing: AAVs engineered for specific tissues allow researchers to direct CRISPR components precisely to the site of interest, minimising off-target effects.
- Multiplexing Capabilities: AAVs can deliver multiple gRNAs simultaneously, making it possible to knock out or modify multiple genes at once—a valuable approach for studying complex genetic traits or diseases.
- In Vivo Applications: Using AAVs to deliver CRISPR components in vivo facilitates gene editing in living organisms, advancing our understanding of gene function and expanding possibilities for therapeutic development.
Through knockout models and gene-editing applications, AAVs combined with CRISPR technology are revolutionising genetic research and paving the way for novel therapeutic strategies.
In Summary
AAVs represent a versatile and promising platform for gene therapy and genetic research. Their unique characteristics, such as long-term gene expression, low immunogenicity, and ability to target a wide variety of tissues, have established them as a cornerstone in the development of novel therapies for genetic disorders, neurological diseases, and beyond.
As research progresses, the integration of AAVs with cutting-edge technologies like CRISPR is enhancing our ability to study gene function and develop effective treatments. However, the challenges associated with pre-existing immunity, transgene capacity limitations, the complexities of scaling AAV production, and potential genotoxicity necessitate careful consideration and ongoing research to optimise AAV applications.
By addressing these challenges and leveraging the strengths of AAVs, scientists and clinicians are poised to unlock new avenues for understanding complex biological processes and developing innovative therapeutic strategies. The future of AAVs in both research and clinical settings is bright, with the potential to transform the landscape of genetic medicine.
References:
- Batty, Paul; Lillicrap, David. Hemophilia Gene Therapy: Approaching the First Licensed Product. HemaSphere 5(3):p e540, March 2021. | DOI: 10.1097/HS9.0000000000000540
- Colella P, Ronzitti G, Mingozzi F. Emerging Issues in AAV-Mediated In Vivo Gene Therapy. Mol Ther Methods Clin Dev. 2017;8:87-104. Published 2017 Dec 1. doi:10.1016/j.omtm.2017.11.007
- Hastie E, Samulski RJ. Adeno-associated virus at 50: a golden anniversary of discovery, research, and gene therapy success–a personal perspective. Hum Gene Ther. 2015 May;26(5):257-65. doi: 10.1089/hum.2015.025. Epub 2015 Apr 20. PMID: 25807962; PMCID: PMC4442590.
- Large, E.E.; Silveria, M.A.; Zane, G.M.; Weerakoon, O.; Chapman, M.S. Adeno-Associated Virus (AAV) Gene Delivery: Dissecting Molecular Interactions upon Cell Entry. Viruses 2021, 13, 1336. https://doi.org/10.3390/v13071336
- Naso, M.F., Tomkowicz, B., Perry, W.L. et al. Adeno-Associated Virus (AAV) as a Vector for Gene Therapy. BioDrugs 31, 317–334 (2017). https://doi.org/10.1007/s40259-017-0234-5
- Wang, JH., Gessler, D.J., Zhan, W. et al. Adeno-associated virus as a delivery vector for gene therapy of human diseases. Sig Transduct Target Ther 9, 78 (2024). https://doi.org/10.1038/s41392-024-01780-w
- Wang D, Zhang F, Gao G. CRISPR-Based Therapeutic Genome Editing: Strategies and In Vivo Delivery by AAV Vectors. Cell. 2020;181(1):136-150. doi:10.1016/j.cell.2020.03.023
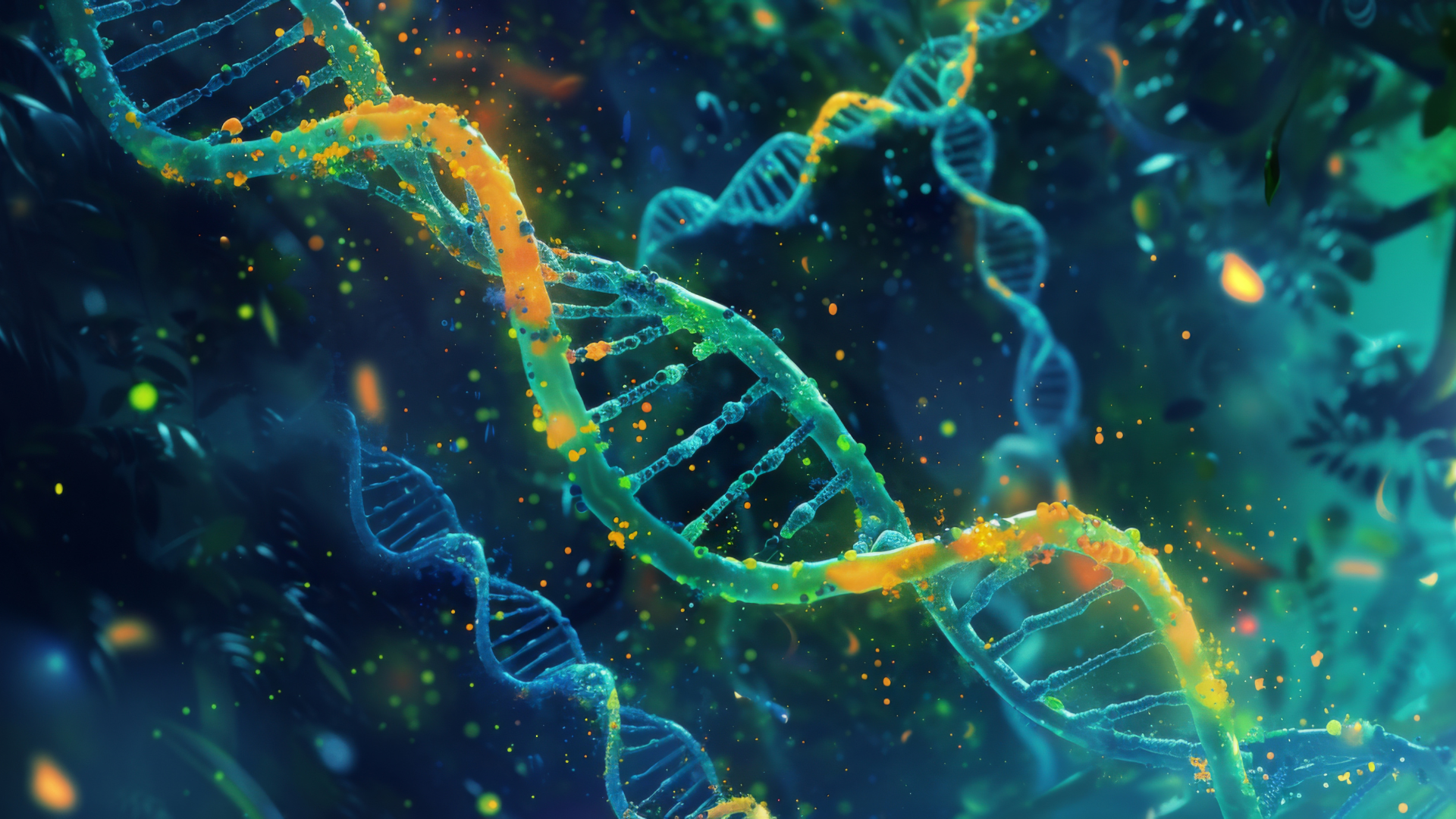