The concept of Critical Quality Attributes (CQAs) plays a pivotal role in a drug development process. According to the ICH Guideline Q8(R2) on Pharmaceutical Development, a CQA is a “physical, chemical, biological, or microbiological property or characteristic that should be within an appropriate limit, range, or distribution to ensure the desired product quality”.
For cell therapies, CQAs encompass various attributes, including purity, identity, cell viability, sterility, transgene integrity and potency.
CQAs are critical to the successful development of cell therapies, ensuring safety, consistency, and therapeutic effectiveness. Each cell therapy product presents unique challenges, requiring rigorous characterization and testing at multiple stages of development. By maintaining well-defined CQAs, manufacturers can enhance product reliability, streamline regulatory approvals, and ultimately improve patient outcomes.
Below, we explore these CQAs for CAR-T cell therapy and mesenchymal stem cells (MSCs)—two distinct but widely used cell therapy platforms.
1. Purity of Cells
As T cell-based therapies continue to evolve, the development of T cell culture media for immunotherapy is crucial for sustaining T cell expansion and activation in a controlled environment. Serum-free T-cell expansion media is especially useful in clinical trials where minimizing serum variability is critical.
For the activation and expansion of T cells, various T cell activation and culture kits are used to standardize the conditions. Another critical consideration is the use of human serum for cell culture or fetal bovine serum alternatives, which provide the essential nutrients for cell growth while maintaining ethical standards in cell production.
According to 21 CFR 610.13, biological products must be free of extraneous material, for example, the absence of undesired cell types, residual impurities, and contaminants in the final cell therapy product.
As part of chemistry, manufacturing, and controls (CMC) development, manufacturers should assess potential contaminants or impurities in the final product during early development.
As the manufacturing process advances, validation efforts should ensure that process-related contaminants are either effectively removed or not introduced. Contaminants must be eliminated, or acceptable limits should be established based on data from product lots that have demonstrated safety in preclinical and/or clinical studies.
Furthermore, a well-defined impurity profile should be developed, detailing any process contaminants and their potential impact on product safety and efficacy.
CAR-T Cells:
- Ensuring that the final CAR-T product consists predominantly of CAR-expressing T cells without excessive contamination by untransduced T cells, NK cells, or other leukocytes.
- Minimizing residual host-cell proteins and nucleic acids from viral vector production for gene editing, as these can pose safety risks.
- Ensuring the absence of undesired genomic mutations or chromosomal abnormalities that may arise during ex vivo expansion.
Mesenchymal Stem Cells (MSCs):
- Ensuring a homogeneous population of CD73⁺, CD90⁺, and CD105⁺ cells while being negative for CD45⁻, CD34⁻, CD14⁻, and HLA-DR⁻ as per ISCT criteria.
- Minimizing contamination with fibroblasts, hematopoietic cells, or differentiated progeny that could compromise MSC functionality.
- Ensuring minimal presence of residual xenogeneic materials (e.g., bovine serum proteins) from culture media used during expansion.
- MSC culture media and proliferation media play vital roles in supporting the growth and maintenance of MSCs and ensuring the cells maintain their ability to proliferate and differentiate as needed.
- Serum-free MSC culture media is often preferred for clinical applications to avoid the variability associated with animal-derived components.
2. Identity Testing of Cells
Identity testing is required throughout all development phases and must be conducted on the final container contents of each lot. This ensures the manufactured cells match the intended cell type and exhibit the expected characteristics while distinguishing them from other products in the facility.
For the initial IND submission, the FDA recommends including:
- A description of the assay, including the antibody panel, gating strategy, and live/dead staining. Relevant cell populations, including non-therapeutic ones (e.g., residual tumor cells), should be documented. A summary of instrument calibration and QC activities should also be provided.
- Written procedures for sample staining, acquisition, and data analysis.
- Antibody titration to optimize dilution.
- Direct detection of CAR expression. If using a surrogate marker, correlation with CAR expression should be evaluated, including sensitivity and specificity.
- A validation study for lot release assays, covering fluorescent marker validation, robustness studies, and sample holding time limits.
CAR-T Cells:
• Flow cytometry to confirm CAR expression on T cells (e.g., CD3⁺/CAR⁺ double-positive population).
• CAR expression analysis via gene detection by PCR.
• CD4/CD8 ratio assessment to ensure an appropriate balance of T cell subsets for effective anti-tumor activity.
• Molecular analysis of CAR transgene integration using qPCR or ddPCR to confirm genetic modification.
MSCs:
- Immunophenotyping to confirm MSC-specific surface markers and exclude hematopoietic contamination.
- Morphological assessment under a microscope to verify plastic adherence and fibroblast-like spindle shape.
- Functional assays to confirm tri-lineage differentiation potential into bone, fat, and cartilage.
3. Cell Viability Test
Viability ensures that a sufficient proportion of cells remain functional and alive at the time of infusion.
- Viability testing (>70%) using Trypan Blue exclusion or flow cytometry (e.g., 7-AAD or Annexin V/PI staining).
- Post-thaw viability assessment, particularly for cryopreserved products, ensuring minimal loss upon thawing.
- Apoptosis assays to detect early signs of cell death that could compromise product efficacy.
4. Sterility Test
The FDA defines sterility as the absence of viable contaminating microorganisms, as outlined in 21 CFR 610.12. Sterility testing typically includes bacterial, viral, fungal, and mycoplasma assays to ensure that cell therapy products are free from harmful contaminants.
The more a cell therapy product is manipulated during manufacturing, the higher the risk of contamination. However, even minimally manipulated products can still carry contaminants. A study of over 4,000 hematopoietic progenitor cell (HPC) products found that more than 1% were contaminated, most commonly with Staphylococcus epidermidis. Despite this, many of these contaminated products were still used in patients due to limited cell availability and urgent clinical needs, with few significant adverse outcomes reported.
Contamination can arise from multiple sources, including:
- Donor-derived contamination
- Manufacturing processes and reagents
- Laboratory equipment (e.g., incubators, water baths, lab benches, and sinks)
- Human handling errors
Regulatory Requirements for Sterility Testing
Sterility testing is a universal quality requirement across all cell therapy categories. For allogeneic cells and tissues, the FDA mandates donor screening for transmissible infections, including HIV-1/2, hepatitis B, hepatitis C, transmissible spongiform encephalopathy, and syphilis. In some cases, additional screening is required for vaccinia, sepsis, West Nile Virus, SARS, and Zika Virus. Leukocyte-rich and reproductive cell donors are subject to further requirements.
Other organizations, such as the International Stem Cell Banking Initiative, recommend additional testing for Epstein–Barr virus, cytomegalovirus, papillomavirus, herpes simplex virus, and other herpes viruses. Notably, autologous donors are exempt from FDA-mandated infectious disease screening.
In-Process Sterility Testing
During manufacturing, in-process sterility testing is essential to detect microbial contamination before product release. Common tests include:
- Sterility testing (USP <71>) – Confirms the absence of bacteria and fungi in the final product.
- Mycoplasma testing – Uses PCR-based assays to detect hidden contamination that could impact patient safety.
- Endotoxin testing using Limulus amebocyte lysate (LAL) assay – Ensures bacterial endotoxins remain within safe limits.
Fungal infections, though rare, typically involve Candida and Aspergillus species, often introduced through medical procedures rather than donor tissues. However, bacterial contamination rates vary significantly by product type—for example, 4.5% of peripheral blood progenitor cell products and 26% of bone marrow harvests have been found to contain bacteria. Studies on allogeneic islet transplants have reported microbial contamination in 16–66% of final preparations, though most cases had minimal clinical impact.
Challenges in Sterility Testing
A major limitation of traditional sterility tests is their long incubation period (14 days), meaning contamination may only be detected after the product has already been administered. To address this, the FDA has approved automated rapid sterility testing systems such as BacT/ALERT and BACTEC, which provide faster results.
Mycoplasma contamination is alarmingly common in cell culture systems, often introduced by personnel handling. More than 50% of cases can be traced back to the human oropharyngeal tract. Because mycoplasma is difficult to eliminate once it enters cells, routine PCR-based mycoplasma screening is crucial.
5. Transgene Integrity
For CAR-T cell therapies, the stability and functionality of the CAR transgene are critical to ensuring therapeutic effectiveness, durability of response, and patient safety. The integrity of the transgene affects CAR expression, persistence, and overall performance of the modified T cells in vivo.
Key Considerations:
- Vector Copy Number (VCN) Assessment
- Measured using quantitative PCR (qPCR) or droplet digital PCR (ddPCR) to determine the average number of CAR transgene copies per genome.
- Ensures consistent gene insertion levels while avoiding excessive integration, which could lead to genotoxicity or altered cell function.
- Regulatory agencies typically set VCN limits to mitigate risks associated with insertional mutagenesis.
- CAR Expression Stability
- Evaluated over multiple cell expansion cycles to ensure that transduced cells retain CAR expression.
- Flow cytometry is commonly used to monitor surface CAR expression levels over time.
- Loss of CAR expression may indicate transgene silencing, epigenetic modifications, or selective depletion of CAR-positive cells, reducing therapy efficacy.
- Off-Target Integration and Genomic Integrity
- Unintended integration sites can disrupt essential genes, leading to potential oncogenic transformation or loss of function in critical pathways.
- Techniques such as whole-genome sequencing (WGS) and integration site analysis are employed to assess insertional mutagenesis risks.
- Non-viral gene delivery methods (e.g., CRISPR/Cas9, transposons) require additional screening for unintended genome edits that could impact safety.
Comparison with MSC Therapies
Unlike CAR-T cells, MSCs are not typically genetically modified for therapeutic applications. Instead, MSCs function via paracrine signalling, immunomodulation, and tissue repair mechanisms. Since MSCs do not rely on stable transgene integration for function, transgene integrity is not considered a relevant CQA for MSC-based therapies.
Ensuring transgene integrity in CAR-T cells is fundamental to maintaining consistent manufacturing processes, predictable in vivo behaviour, and long-term safety for patients.
6. Potency of Cell
Potency is a fundamental quality attribute in cell therapy, confirming that a product has a biological function relevant to treating the intended condition. The FDA defines potency testing as a means to ensure that only product lots meeting specific criteria are used in clinical development and post-market approval. Best practices involve selecting potency assays that align with the therapy’s expected mechanism of action, linking them to in vivo functions and clinical efficacy.
Developing reliable potency assays for cell therapies presents unique challenges. Unlike identity, purity, and sterility tests, potency assessments lack standardized regulatory guidelines, requiring customized assays for each product. In cases where the mechanism of action (MOA) is unknown, the number of viable cells often serves as a surrogate potency measure. However, even when MOA is well understood, in vitro potency assays frequently fail to predict in vivo efficacy. This discrepancy arises because biological function often depends on the in vivo microenvironment. For example, glucose-stimulated insulin secretion in vitro does not consistently correlate with islet transplant outcomes, just as in vitro cytotoxicity of T cells against cancer cells does not always predict in vivo potency in certain CAR-T therapies.
The FDA acknowledges several challenges in potency testing for cell therapies, including variability in starting materials, small lot sizes, limited stability, lack of reference standards, multiple active components, and potential interactions between them. A recent FDA review found that fewer than half of submitted INDs included any bioactivity assay, highlighting the need for robust potency evaluation early in development.
Ensuring Product Potency and Consistency in Manufacturing
To maintain potency throughout development and commercialization, potency testing must be integrated into manufacturing:
- Potency testing is required before pivotal clinical trials, but in vitro potency data should ideally be collected early in development. This is especially crucial for rare diseases, where early clinical studies may serve as registrational trials, making it essential to establish potency assays as soon as possible.
- The manufacturing process should be designed and validated to maintain the product’s potency and ensure consistency across different production lots.
- The quality of raw materials used in manufacturing can impact product potency. Therefore, all materials should meet predefined specifications before use.
- Containers, closures, and equipment that come into direct contact with the product should be assessed for any potential adverse effects on potency.
- Adequate manufacturing controls and in-process testing should be implemented to maintain product potency.
- Potency assays used for lot release must be verified for their intended purpose, ensuring sufficient specificity, accuracy, and precision over the assay’s reportable range. Their performance characteristics should be established and documented during assay qualification and validation.
- Phase-appropriate potency assays and acceptance criteria should be defined, and any production lots that fail to meet these criteria should be rejected.
CAR-T Cells:
- Evaluated by cytotoxicity assays to confirm that CAR-T cells specifically recognize and kill target cancer cells.
- Assessing cytokine secretion profiles (e.g., IFN-γ, TNF-α, IL-2) upon antigen stimulation to ensure robust immune activation.
- Evaluating proliferation capacity after antigen engagement, as expansion in vivo is critical for sustained therapeutic effects.
MSCs:
- Functional assays measuring immunosuppressive properties, such as MSC-mediated inhibition of T cell proliferation.
- Evaluating secretion of anti-inflammatory cytokines (e.g., TGF-β, IL-10, PGE2) that contribute to immunomodulation.
- Differentiation assays confirm the ability of MSCs to form osteogenic, adipogenic, and chondrogenic lineages, ensuring their regenerative potential.
Quality Target Product (QTPP)
A QTPP should provide a summary of the product’s potency-related characteristics. It should be developed based on an understanding of the product’s mechanism of action (MOA), intended clinical indication, and route of administration.
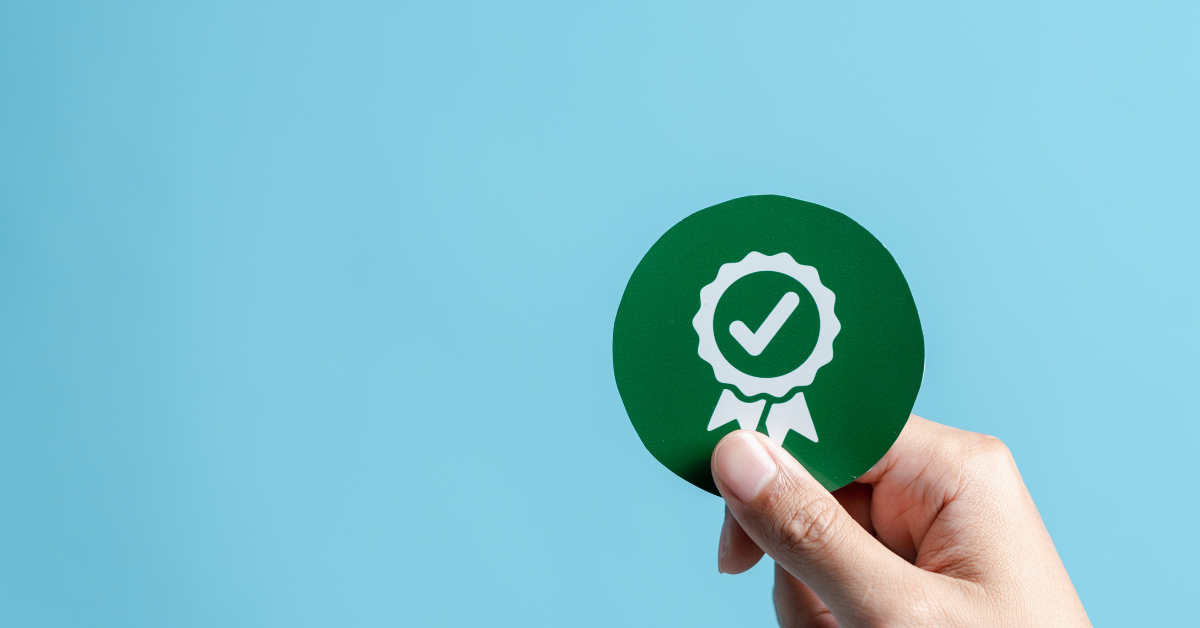
Key Considerations for Product Development
Mechanism of Action (MOA)
Understanding the MOA is essential for identifying potency-related critical quality attributes (CQAs) and developing a potency assurance strategy. If the MOA is not fully understood, efforts should continue throughout development to refine this knowledge, updating potency-related CQAs and the potency assurance strategy accordingly. Cell and gene therapy (CGT) products often exhibit multiple biological activities, and the most relevant activities may vary depending on the targeted disease. The intended clinical indication should be considered when determining which quality attributes are critical to product potency.
Nonclinical Studies
Early-stage nonclinical studies can provide insights into the product’s MOA and help establish links between product attributes and therapeutic effects. Data from these studies should inform the initial potency assurance strategy, including the selection of potency-related CQAs and appropriate acceptance criteria.
Product Characterization and CQA Identification
Comprehensive product characterization, starting from early development, helps in understanding product attributes and identifying potency-related CQAs. Characterization assays do not necessarily need to be qualified but should be scientifically sound, precise enough to detect meaningful differences, and reliable for their intended purpose. Additionally, characterization data can be useful in assessing the impact of manufacturing changes.
Establishing a Relationship Between CQAs and Potency
Ideally, potency-related CQAs should have a well-defined link to the product’s MOA, supported by prior knowledge, product characterization, and nonclinical, or clinical studies. In cases where the MOA is not fully understood, statistical correlations between product attributes and nonclinical or clinical outcomes may indicate relevance to potency. However, statistical associations alone are insufficient to confirm a mechanistic relationship and further experimental validation may be required.
Impact of Material Quality on Potency
During manufacturing development, it is important to assess whether raw material attributes influence potency-related CQAs. This information supports material specification development, supplier qualification, and supply chain risk management.
Establishing and maintaining well-defined CQAs is essential for ensuring the safety, efficacy, and consistency of cell therapy products. The complexity of these therapies, particularly CAR-T cells and MSCs, requires rigorous characterization at multiple stages of development. By implementing robust analytical assays and quality control measures for purity, identity, viability, sterility, and transgene integrity, manufacturers can optimize product reliability and regulatory compliance. Advances in rapid sterility testing, genomic integrity assessments, and potency evaluations continue to refine the CQA framework, facilitating the development of safer and more effective cell-based therapies. Ultimately, a comprehensive understanding and control of CQAs not only supports regulatory approval but also enhances patient outcomes in the evolving landscape of cell therapy.
References
- Dominici et al. Minimal criteria for defining multipotent mesenchymal stromal cells. The International Society for Cellular Therapy position statement. Cytotherapy. (2006)
- Karanu, et al. (2020). Improved harmonization of critical characterization assays across cell therapies. Regenerative Medicine, 15(5), 1661–1678.
- International Council for Harmonisation (ICH). Pharmaceutical Development Q8(R2) – Guideline on Quality by Design.
- Mendicino et al (2014). MSC-based product characterization for clinical trials: an FDA perspective. Cell Stem Cell, 14(2), 141–145. https://doi.org/10.1016/j.stem.2014.01.013
- US FDA Guidance for Industry: Potency Tests for Cellular and Gene Therapy Products. Center for Drug Evaluation and Research (CDER) (2011).
- US FDA CMC Considerations for Early-Phase Studies of Cell and Gene Therapy Products – Regulatory Do’s and Don’ts: Tips from FDA Staff. Office of Cellular Therapy and Human Tissue CMC, Office of Therapeutic Products. (2024).
- US FDA Guidance for Industry: Potency Assurance for Cellular and Gene Therapy Products (2023).