Table of Contents
- Inherent manufacturing challenges faced in cell and gene therapies
- Strategies for Scaling Up Cell and Gene Therapies
- Examples of Automated Platform in Cell Therapy Manufacturing
- Key Takeaways and Future Directions in Scaling Cell and Gene Therapy Manufacturing
Cell and gene therapies are at the forefront of modern medicine, offering the potential to cure a wide range of diseases. Expanding these therapies from laboratory research to large-scale commercial production is essential for broad patient access. However, this scaling process presents significant challenges, particularly in preserving quality and efficacy while also ensuring cost-efficiency.
Inherent manufacturing challenges faced in cell and gene therapies
- Predominantly manual and labor-intensive, making processes prone to human error.
- Often involve open systems that are difficult to scale and heavily dependent on the operator’s experience and judgment.
- Risk of contamination and potential batch loss.
- For autologous products, which rely on patient or donor cells as the starting material, there is a significant potential for batch-to-batch variation due to the inherent complexity of biological products. The patient’s own cells are used in the manufacturing process. Therefore, the quality of the therapeutic product is directly influenced by the quality of the donor cell material. Patients who are older or suffering from severe illnesses often have “exhausted” cells, which are less responsive to stimulation. This cellular exhaustion can result in weaker immune responses and, consequently, a less effective therapeutic outcome.
Manufacturing scalability must be considered early in the development of cell and gene therapies.
A key challenge in developing and commercializing cell and gene therapies is scaling up production, especially as companies move from clinical trials to full-scale manufacturing. Achieving scalability without compromising quality is critical, as failure can lead to significant financial losses and product failure.
- Manufacturers must balance increasing batch sizes with maintaining consistency and meeting regulatory requirements, which complicates changes to the process later on.
- Regulatory bodies require thorough documentation of the production processes making it difficult to alter manufacturing processes later in the commercialisation process.
- Investors are also increasingly evaluating whether companies have well thought-out plans for scaling up and commercialisation
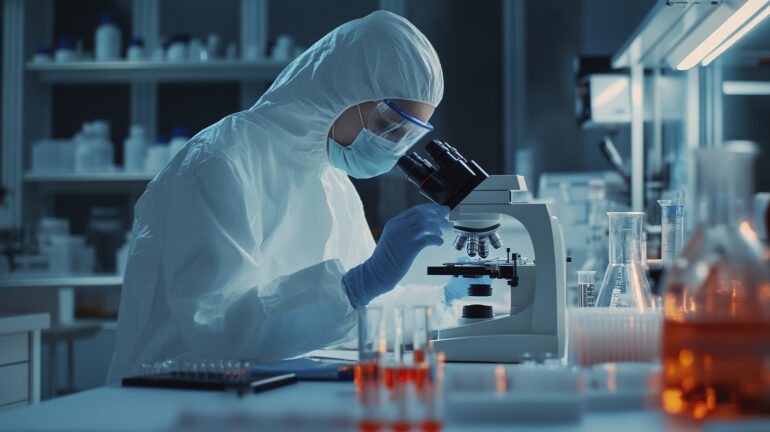
Strategies for Scaling Up Cell and Gene Therapies
1) Continuous monitoring and process optimisation can lead to more efficient production processes.
Key Considerations for Effective Process Development for T-cell Therapies:
- Purification of the Starting Material
Removing unwanted cell populations from the collected material ensures consistency and eliminates potentially suppressive cells, such as circulating blasts in conditions like acute myeloid leukemia (AML).
Advanced technologies such as CliniMACS and Prodigy from Miltenyi facilitate the enrichment of these desired T-cell populations and the depletion of unwanted subsets, ensuring that the process is conducted in a manner compatible with Good Manufacturing Practices (GMP).
Enhancements to the engineered T-cell products can be made by using specific T-cell subsets, such as CD4+/CD8+ and CD62L+ (naïve and central memory T cells). This selection improves the function and persistence of the cells when they are reintroduced into the patient.
- T-cell Activation
Activation of T cells is a pivotal step in the manufacturing process of genetically modified T cells, as it initiates the necessary cellular processes for robust and rapid ex vivo expansion to achieve a sufficient yield of functional T cells, which are crucial for therapeutic applications in clinical settings.
Activation Methods: T-cell activation can be accomplished using various reagents targeting CD3 and CD28 receptors. Historically, methods such as using magnetic beads coated with anti-CD3 and anti-CD28 antibodies have been employed to both isolate and stimulate T cells. However, the removal of these beads is required, adding complexity to the manufacturing process.
Alternative Activation Technologies: There are soluble activation reagents that simplify the process by eliminating the need for bead removal, as well as novel technologies like nanomatrix-based and hydrogel platforms that offer additional options for T-cell activation.
Optimization Parameters: Regardless of the activation method chosen, critical parameters such as seeding density, the type of activation vessel, and the duration of activation must be carefully optimized. Overexposure to activation signals can lead to an overly differentiated and exhausted T-cell product, which could negatively impact the therapy’s effectiveness.
- Expansion
Key considerations for the process development at the cell expansion step for T-cell therapies include the selection of suitable expansion media, oxygen supply, gas exchange, and the appropriate expansion platform.
Expansion Media: The choice of T-cell expansion media is crucial. While early-phase clinical trials may use serum-supplemented media, serum-free media is preferred for later phases due to its reduced risk of pathogen contamination and more consistent product quality.
Oxygen Supply and Gas Exchange: Efficient oxygen supply and gas exchange are essential for the expansion of activated and transduced T cells. New-generation culture devices, such as gas-permeable flasks and bioreactors, offer scalable solutions for producing large quantities of immune cells.
Scalable Expansion Platforms: Platforms like G-Rex devices and bioreactors provide scalable, closed-system processing, which is important for large-scale manufacturing. These systems allow for automated media exchange and efficient waste removal, supporting the expansion of various immune cell types.
Key Considerations for Effective Process Development for Human Mesenchymal Stem Cells (hMSCs)
- Large-Scale Cell Expansion
For scalable hMSC expansion, alternative bioreactors such as multi-layered cell factories, roller bottles, hollow fiber systcems, packed beds, and stirred suspension bioreactors are available.
Scaling up bioreactor cultures involves managing oxygen supply and hydrodynamic shear. Hydrodynamic shear, controlled by impeller agitation, needs careful balancing to prevent cell damage or poor mixing. Tools like computational fluid dynamics and particle image velocimetry are useful for identifying optimal parameters for scaling up.
There are 2 key bioreactor types used for hMSC expansion:
Multi-Layered Vessels
- simplify scaling up from monolayer cultures by providing extensive surface area through stacked layers of ‘flask-units’ (e.g. FD Cell Factory) to obtain clinical doses of hMSCs (>200 × 10^6 cells).
- These systems face challenges in monitoring and uniform cell distribution, potentially leading to increased culture heterogeneity.
Stirred Suspension Bioreactors
- have a centrally located impeller that ensures uniform mixing
- can operate in batch, fed-batch, or perfusion modes.
- reduce vessel-to-vessel variability and labor costs, with options for single-use, closed bioreactors
- Support high cell densities by using microcarriers, which offer a large surface area for cell attachment and growth. These microcarriers, available in materials like collagen, dextran, and glass.
- Key factors include microcarrier selection, loading density, and medium composition, all crucial for high cell yields and consistent culture performance.
- Cell detachment and separation
During the scale-up of MSC manufacturing, cell detachment and separation present significant challenges. After cultivation, cells are detached from microcarriers using proteolytic enzymes like trypsin or a combination with collagenase if biodegradable carriers are used. The process must be optimized to minimize enzyme exposure and ensure high cell viability. Mechanical agitation can aid detachment without compromising cell quality.
After detachment, cells are separated from the microcarriers, typically using size exclusion filtration. At small scales, sterile sieves or filtration devices like Steriflip® are used.
For larger scales, systems like Harvestainerâ„¢ BioProcess Containers are employed, ensuring high cell recovery and minimal contamination. Throughout the process, maintaining cell viability and preventing microcarrier debris from contaminating the final product are critical considerations.
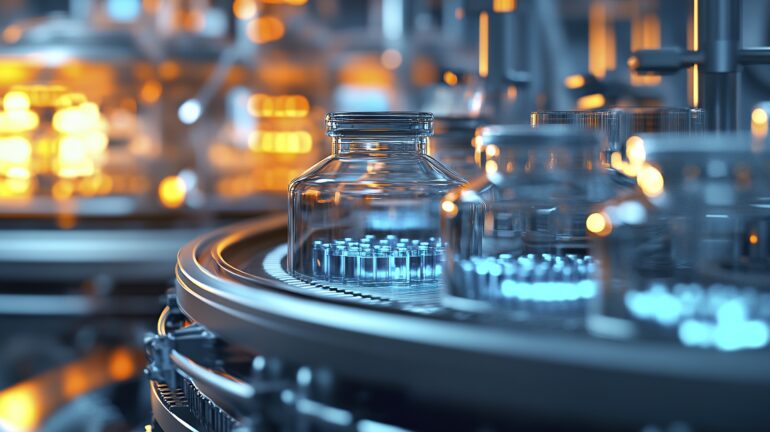
2) Benefits of Implementing Automation in Cell Therapy Manufacturing
Automation in cell therapy manufacturing can increase scalability, reduce contamination risk, and enhanced process consistency.
- Improved Reliability and Reproducibility
Automation can reduce in-process variation by using robotic arms to consistently perform tasks like pipetting, mixing, or even entire cell culture sub-processes (e.g., medium changes) with precise speed, force, and accuracy, resulting in less variability and greater process reliability.
- Protection from Contamination
Automation can replace manual operations, like cell culture and quality control, with closed systems that protect the cell product from both environmental contaminants and operator error. These systems not only improve the reliability and reproducibility of the manufacturing process but also facilitate process monitoring and control, which are critical for maintaining product quality.
- Enhanced Process Monitoring and Control
Automation can provide more control over a bioprocess through the use of sensors that produce online and continuous measurements, while leading to a more accurate and faster process optimization.
- Reduced Human-Induced Variability
Besides biological variation which is difficult to tackle due to the complexity of these products, in-process variation occurring from human handling is another persistent issue that can impact product quality. Even when stringent protocols are used, variation is observed between different handlers as a result of minor imprecisions in protocols (e.g. slight deviations in incubation times, variation in pipetting etc.)
- Precise Parameter Control
Exact settings and timing can be saved and tracked, improving process consistency.
- Objective Decision-Making
Automation integrates analytics, eliminating subjectivity in processing decisions like determining cell confluence. For instance, pattern recognition and image processing software can objectively assess confluence, replacing subjective estimates based on microscopic visualisation. This automation allows for adaptive processing based on clear, objective criteria.
- Increased Throughput
automation can be adapted for increased throughput/parallelisation of systems and it offers an enhanced possibility to monitor and track data.
Challenges of Implementing Automation in Cell Therapy Manufacturing
- Limited Standardization
The diversity of cell types and processes limits standardized, off-the-shelf automation solutions, leading to developers relying on custom-designed equipment, which increases cost and time for implementation.
Potential Solution:
Invest in modular automation platforms that can be customized and adapted to different cell types and processes. Collaboration between developers and equipment manufacturers can also lead to the creation of more versatile systems.
- Process Understanding
Effective automation requires a clear understanding of critical process parameters (CPPs) and critical quality attributes (CQAs), which guide the design of user requirements and operational parameters to ensure consistency and product quality.
Potential Solution:
A strategic approach, including thorough process mapping and knowledge gathering, is essential. This involves creating detailed process flow diagrams with CPPs, CQAs, and a control strategy based on criticality analysis to define specific automation equipment requirements.
- Timing Challenges
Introducing automation too early in the product development lifecycle can hinder process flexibility, while delaying automation may require process changes that could affect product comparability, particularly if the product has already undergone clinical testing.
Potential Solution:
Adopt a phased approach to automation, where initial process development is done manually to allow flexibility, followed by gradual integration of automation as the process becomes more defined. Implement pilot automation systems early to test and refine the process before full-scale automation is introduced, ensuring product comparability.
- Operational Considerations
Companies must align automation technologies with the specific operational needs of their manufacturing process, considering factors like process duration, scale, and operation type. Small-scale processes might benefit from multi-functional equipment, while larger-scale processes may require modular systems for flexible scaling.
Potential Solution:
Carefully evaluate the specific needs of the manufacturing process, such as scale and duration, when selecting automation equipment. For small-scale operations, consider multi-functional equipment, while larger-scale processes might benefit from modular systems that allow for easy scalability. Tailor the automation strategy to the specific phase of production, balancing flexibility with efficiency.
- GMP Biomanufacturing Compliance
Ensuring automation equipment is fit for purpose within a good manufacturing practice (GMP) environment requires a rigorous qualification process, including installation qualification (IQ), operational qualification (OQ), and performance qualification (PQ), to confirm that the equipment meets the product and process needs.
Potential Solution:
Implement a rigorous qualification process for automation equipment, including installation qualification (IQ), operational qualification (OQ), and performance qualification (PQ). Involve cross-functional teams, including quality assurance and regulatory experts, in the qualification process to ensure that the equipment meets GMP requirements and is fit for purpose. Regularly review and update qualification procedures to adapt to evolving regulatory standards.
Examples of Automated Platform in Cell Therapy Manufacturing
1st generation platforms improved consistency in cell culture, while 2nd generation systems offer complete automation, flexibility, and enhanced control, making them ideal for advanced cell and gene therapy production.
1st Generation Platforms:
- Addressed inconsistencies in manual cell culturing using robotic arms and pipetting robots for improved accuracy and reproducibility.
- Systems like CompacT SelecTâ„¢ and Cellmate (Sartorius) enabled processing of larger volumes and were adopted for large-scale stem cell manufacturing and viral vectored gene therapies.
- Additional systems, such as CyBio®, RoboLector, and Biomek® 4000, automated tasks but requires additional equipment and are limited by large footprints, high costs, and reliance on specific consumables and operator skills.
2nd Generation Platforms:
- Introduced complete automation by integrating multiple operational units into closed systems, reducing contamination risks and potentially eliminating the need for clean rooms.
- Examples include CliniMACS Prodigy (Miltenyi Biotech), Cocoonâ„¢ (Octane Biotech), and Quantumâ„¢ (Terumo BCT), which offer continuous process validation and monitoring.
- Newer systems like AUTOSTEM offer modular, fully automated solutions for cell therapy manufacturing, handling everything from tissue collection to cryopreservation in a single platform.
Key Takeaways and Future Directions in Scaling Cell and Gene Therapy Manufacturing
Scaling up cell and gene therapies from laboratory research to large-scale commercial production involves navigating significant challenges. The transition requires extensive modifications in equipment, protocols, and quality assurance measures, including moving from manual, lab-based processes to sophisticated bioreactors and automation systems. Key challenges include maintaining quality and consistency while balancing increasing batch sizes and meeting regulatory requirements. Automation plays a critical role in addressing these challenges by enhancing process reliability, reducing contamination risks, and improving process control.
As the industry progresses, there is a shift towards greater automation, driven by the need for efficiency and scalability. While current diversity in cell types and processes limits the development of standardized automation platforms, the increasing commercialization of therapies may reveal commonalities that could lead to more universally applicable automation solutions. These advancements are expected to reduce costs, streamline manufacturing processes, and improve patient access to advanced therapies. Thus, a strategic approach to scaling up, incorporating thorough process mapping and modular automation, is essential for successful commercialization and widespread application of cell and gene therapies.
References:
- Panchalingam KM, Jung S, Rosenberg L, Behie LA. Bioprocessing strategies for the large-scale production of human mesenchymal stem cells: a review. Stem Cell Research & Therapy. 2015
- Moutsatsou P, Ochs J, Schmitt RH, Hewitt CJ, Hanga MP. Automation in cell and gene therapy manufacturing: from past to future. Biotechnology Letters. 2019
- https://www.biopharminternational.com/view/addressing-cell-therapy-challenges-through-a-modular-closed-and-automated-manufacturing-system
- https://www.bioprocessintl.com/cell-therapies/automation-in-cell-therapy-manufacturing