Mesenchymal stem cells (MSCs) hold immense potential in regenerative medicine due to their ability to differentiate and their immunomodulatory properties. Cryopreservation, the storage of cells at ultra-low temperatures, is crucial for maintaining a readily available supply of MSCs for therapy. However, the process itself presents significant challenges.
In this blog, we will delve into the key challenges associated with cryopreservation of
MSCs and explore potential solutions.
Why MSCs Biobanking is Essential for Future Therapies
Cryopreserving MSCs and creating an MSC biobank offers a strategic approach to ensuring a readily available supply of high-quality cells for regenerative medicine applications. It allows for standardisation and reduces dependence on fresh cell isolation. There are several compelling reasons why cryopreserving MSCs and creating a biobank is important for advancing regenerative medicine:
- Readily Available Cells: MSCs are often isolated from tissues like bone marrow or fat. Having a cryopreserved supply in a biobank eliminates the need to obtain fresh cells for each treatment, saving time and reducing patient discomfort. This is especially crucial for situations requiring immediate cell therapy.
- Standardised Quality: Cryopreservation allows for the creation of a bank of MSCs with well-defined characteristics. These cells can be rigorously tested and characterised for their therapeutic potential before being cryopreserved. This ensures a consistent and reliable source of high-quality MSCs for clinical use.
- Off-the-Shelf Therapies: Biobanks of cryopreserved MSCs can facilitate the development of “off-the-shelf” cell therapies. These therapies wouldn’t require matching a specific donor to a recipient, overcoming limitations associated with traditional cell therapy approaches.
- Reduced Donor Variability: MSCs obtained from different donors can exhibit variations in their properties and therapeutic effects. A biobank allows researchers and clinicians to select cells with the most desirable characteristics for specific applications.
- Long-Term Storage: Cryopreservation allows for long-term storage of MSCs. This is valuable for research purposes, preserving valuable cell lines for future studies, and potentially enabling the creation of cell therapies for future generations.
- Sharing and Collaboration: Biobanks can facilitate the sharing of MSCs between research institutions and companies. This promotes collaboration and accelerates the development of new cell-based therapies.
Cryopreservation Challenges of MSCs
Cryopreservation stands as a pivotal technique in regenerative medicine, ensuring the availability of MSCs for therapeutic applications. However, within this crucial process lie formidable challenges that researchers and clinicians must navigate. From the delicate balance between cryoprotectant toxicity and efficacy to maintaining stemness amidst freezing and thawing, the journey of preserving MSCs is fraught with hurdles.
- Reduced Cell Viability: During freezing, water in the cell can form ice crystals, which can puncture the cell membrane and damage internal structures, leading to cell death. The removal of water during freezing causes changes in the concentration of solutes inside the cell which lead to osmotic stress causing cells to shrink and damaging the cellular components.
- Cryoprotectant Toxicity: Cryoprotective Agents (CPAs) such as dimethyl sulfoxide (DMSO), glycerol (GLY), ethylene glycol (EG), or propylene glycol(PG) are essential as they help reduce ice crystal formation and protecting cell membranes. While essential, CPAs can be cytotoxic at high concentrations. Finding the optimal balance between cryoprotection and cytotoxicity is crucial.
- Loss of Stemness: The ECM provides vital cues for MSCs to maintain their stemness and differentiation potential. Cryopreservation can disrupt these interactions, potentially affecting the ability of MSCs to self-renew and differentiate into specialised cell types. Freezing and thawing can alter the epigenetic landscape of MSCs, which are chemical modifications on DNA that regulate gene expression. These changes can affect the expression of genes important for stemness and differentiation.
- Standardisation Issues: Cryopreservation protocols vary significantly across labs, with differences in freezing/thawing rates, cryoprotectant concentrations, and cell culture conditions. This inconsistency can lead to variable results in terms of cell viability, stemness, and overall functionality of cryopreserved MSCs. The lack of standardised quality control measures makes it difficult to compare MSCs cryopreserved using different protocols. Establishing robust quality control procedures is essential for ensuring the safety and efficacy of cryopreserved MSCs for clinical use.
Important Parameters for Cryopreservation of MSCs
As the freezing process can impact the viability and functionality of these cells, it’s crucial to evaluate several key parameters to ensure successful cryopreservation of MSCs to ensure that cryopreserved MSCs retain their therapeutic potential for future applications.
- Immunomodulatory Ability: This refers to the ability of MSCs to modulate the immune system. A critical aspect is their immunosuppressive effect on lymphocytes or peripheral blood mononuclear cells (PBMCs). Assays can measure cytokine secretion or T-cell proliferation after co-culture with MSCs.
- Cell Differentiation Capability: MSCs have the potential to differentiate into various cell types. This can be assessed using specific staining techniques:
- Oil Red O: Detects lipid accumulation, indicating differentiation towards adipocytes (fat cells).
- Alizarin Red: Stains calcium deposits, a marker for osteoblast differentiation (bone cells).
- Alcian Blue: Identifies glycosaminoglycans, present in chondrocytes(cartilage cells).
- Cell Viability: It is important to measure the proportion of living cells after cryopreservation. Common assays include:
- Annexin V-Propidium Iodide (Annexin V-PI): Differentiates between viable, apoptotic (dying), and necrotic (dead) cells based on membrane integrity and phosphatidylserine exposure.
- Live-Dead Cell Staining (e.g., Calcein AM/Ethidium homodimer): Live cells take up the green fluorescent dye (calcein AM), while dead cells stain red with ethidium homodimer
- Cell Phenotype and Proliferation Capacity: Specific surface markers define MSCs. They should express CD90, CD105, and CD73, but lack markers associated with hematopoietic stem cells, such as CD34, CD19, CD45, andCD14. Flow cytometry is commonly used to assess these markers. Proliferation capacity can be determined by cell counting assays or measuring DNA synthesis.
- Biosafety and Homing/Engraftment Potential: Cryopreservation shouldn’t introduce genetic mutations or increase the risk of tumour formation or the ability of MSCs to migrate to the site of injury. Preclinical in vivo animal studies can evaluate these risks.
Strategies for Successful MSC Cryopreservation
As researchers strive to ensure the viability and functionality of cryopreserved MSCs, they encounter a spectrum of challenges. Yet, amidst these challenges, researchers are looking into innovative solutions to optimise the cryopreservation process:
- Workspace and Reagent Preparation: Before beginning the cryopreservation process, it is essential to ensure that your workspace and all reagents are prepared. Decontaminate surfaces with mycoplasma spray or other suitable disinfectants to maintain a sterile environment. Have all necessary reagents, including cryoprotectants and growth media, ready and chilled if required. This preparation minimises the time cells are exposed to suboptimal conditions, enhancing their chances of survival during the freezing and thawing processes.
- Precision Cooling: A key factor is the cooling rate. Controlled-rate freezing before long term storage maximises cell viability. Slower freezing with controlled temperature gradients minimises ice crystal formation, which can damage cells. If a specialised freezer isn’t available, consider using a thermally controlled container such as an alcohol-free freezing container that cools at a stable rate of -1℃/minute. It’s important to ensure that MSCs are frozen at the recommended density, typically between 5 x 10^5 cells/mL and 1 x 10^6 cells/mL, to maintain their integrity during the freezing process. Additionally, always use sterile cryovials to store cells, ensuring that they are properly labelled and securely sealed before beginning the cooling process.
- Temperature Matters: Liquid nitrogen storage at -196°C is considered optimal. At this ultra-low temperature, cells enter a state of metabolic stasis, minimising cellular activity and the risk of damage. For shorter storage periods (a few months), -80°C may be acceptable. However, for long-term cryopreservation (over a year), -196°C is strongly recommended. Crucially, maintaining a consistent temperature throughout storage, including during transport or sample retrieval, is vital. Thermal fluctuations can significantly affect cell health upon thawing.
- Rapid Thawing: The rate of rewarming cryopreserved samples impacts cell viability, though generally less critically than the controlled cooling phase. The most common approach involves a water bath at 37°C until all ice crystals disappear. This rapid thawing minimises exposure to potentially damaging intracellular ice formation.
- Post-Thaw Revival Strategies: After thawing, removing cryoprotectants with a dilution step using prepared growth medium followed by centrifugation is essential. Additionally, providing the right culture conditions with essential growth factors helps thawed MSCs recover, regain functionality, and thrive.
- Balancing Protection and Toxicity: Cryoprotectants, like the commonly used DMSO-containing freezing media, are crucial for preventing freezing damage and ensuring cell viability upon thawing. However, the paradox arises in their potential cytotoxicity, particularly at elevated concentrations. To mitigate this risk, researchers are exploring alternative formulations, such as employing lower concentrations of DMSO in conjunction with non-cytotoxic biocompatible substances. For instance, a blend of 5% DMSO with 95% human serum albumin has shown promise in maintaining cell integrity while minimising cytotoxic effects. Moreover, ongoing research endeavours aim to pioneer novel cryoprotectants harnessing the power of natural biomaterials, heralding a safer approach that may even obviate the need for DMSO entirely.
- Encapsulation for Enhanced Protection: Encasing MSCs in protective hydrogels or other biomaterials during cryopreservation can provide an extra layer of defense against cellular stress. Promising advancements include freezing cells encapsulated in nanoliter droplets on highly hydrophobic nano-rough surfaces and the development of nontoxic, nanoscale bio-inspired cryoprotectants.
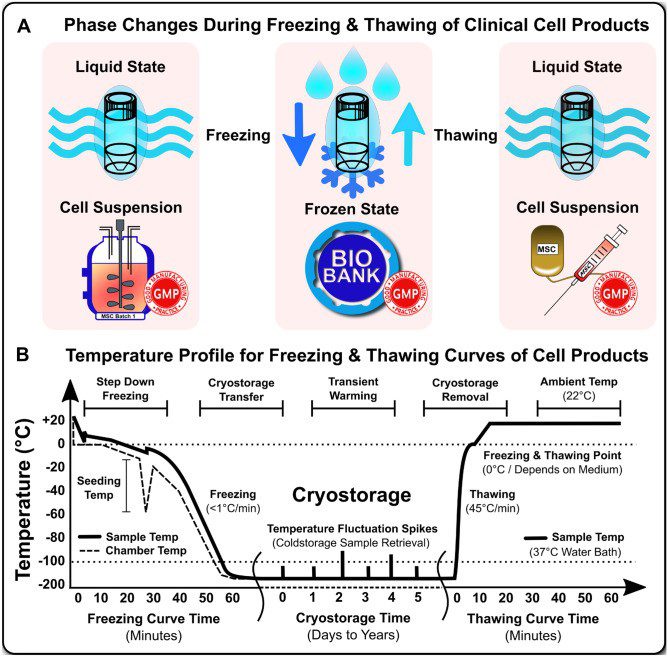
Figure 1: Cryopreservation and freeze-thawing of cell products. (A) Phase changes during freezing and thawing of clinical cell products. (B) Temperature profile for freezing and thawing curves of cell products.
Credit: Cottle, C., Porter, AP., et. al., doi: 10.1007/s40778-022-00212-1
Reproduced under the Creative Commons license
Conclusion:
Despite the challenges, cryopreservation remains a vital tool for advancing MSC-based therapies. Developing standardised cryopreservation protocols across labs is essential. Robust quality control measures ensure consistent and safe results, paving the way for reliable clinical applications of cryopreserved MSCs.
References:
Cottle C, Porter AP, Lipat A, et al. Impact of Cryopreservation and Freeze-Thawing on Therapeutic Properties of Mesenchymal Stromal/Stem Cells and Other Common Cellular Therapeutics. Curr Stem Cell Rep. 2022;8(2):72-92. doi:10.1007/s40778-022-00212-1
Diaferia GR, Dessì SS, Deblasio P, Biunno I. Is stem cell chromosomes stability affected by cryopreservation conditions? Cytotechnology. 2008 Sep;58(1):11-6. doi: 10.1007/s10616-008-9163-y. Epub 2008 Oct 8.
Guven S, Demirci U. Integrating nanoscale technologies with cryogenics: a step towards improved biopreservation. Nanomedicine (Lond). 2012 Dec;7(12):1787-9. doi: 10.2217/nnm.12.158.
Li CH, Zhao J, Zhang HY, Wang B. Banking of perinatal mesenchymal stem/stromal cells for stem cell-based personalized medicine over lifetime: Matters arising. World J Stem Cells. 2023;15(4):105-119. doi:10.4252/wjsc.v15.i4.105
Linkova DD, Rubtsova YP, Egorikhina MN. Cryostorage of Mesenchymal Stem Cells and Biomedical Cell-Based Products. Cells. 2022 Aug 29;11(17):2691. doi: 10.3390/cells11172691.
Yong KW, Wan Safwani WK, Xu F, Wan Abas WA, Choi JR, Pingguan-Murphy B. Cryopreservation of Human Mesenchymal Stem Cells for Clinical Applications: Current Methods and Challenges. Biopreserv Biobank. 2015;13(4):231-239. doi:10.1089/bio.2014.0104