Extracellular vesicles (EVs) have emerged as a captivating area of research in recent years. These tiny, lipid membrane-bound structures that are released by most cells, act as natural communication channels, shuttling biomolecules and information between cells. Their potential for therapeutic applications and as drug delivery carriers is vast, owing to their inherent ability to deliver cargo within the body and to shield the cargo from degradation.
Despite their promise, developing high-quality, scalable EVs is challenging. However, engineered EVs represent a new frontier. In this blog post, we delve into the world of both native and engineered EVs, exploring their characteristics, limitations, and the exciting possibilities that engineered EVs hold for the future of medicine. We will discuss the advantages and challenges of each approach, highlighting the potential of this rapidly evolving field to revolutionise targeted therapies.
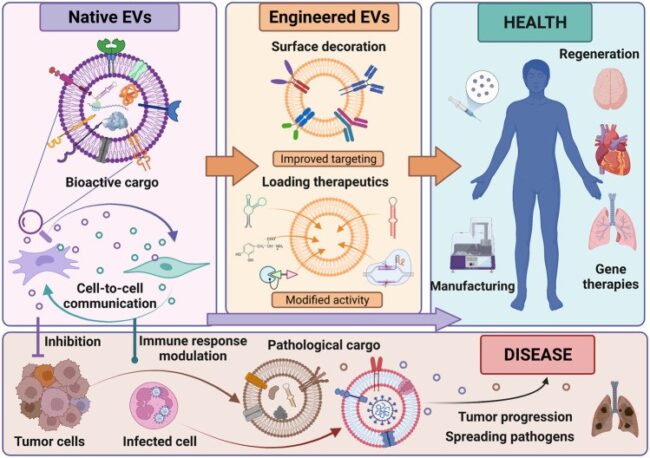
Figure 1: Characteristics, bioengineering, and role of EVs in health and disease. EVs carry bioactive cargo such as proteins, lipids, and nucleic acids that influence target cells. They can be bioengineered through surface modifications or by loading specific therapeutic cargo to enhance their functionality and specificity. Both native and engineered EVs hold promise as next-generation cell-free therapeutics. However, EVs released by cancer or infected cells contribute to disease progression and spreading.
Credit: Bobis-Wozowicz S, Marbán E, doi. 10.3389/fcell.2022.919426
Reproduced under the Creative Commons license
Native EVs: Nature’s Endogenous Messengers
Native EVs represent a fundamental communication pathway within multicellular organisms. They are naturally released by cells without undergoing any modifications to either the EVs or the cells that secrete them. They encapsulate a diverse repertoire of biomolecules, including proteins, lipids, and nucleic acids. Membrane proteins include tetraspanins (CD9, CD63, CD81, etc), adhesion molecules (integrins, EpCAM, Ephrin, etc.), MHC, and receptors. Nucleic acids include DNA and RNA (mRNAs, miRNAs, LncRNAs, and circRNAs). These biomolecules effectively reflect the functional state of their originating cells.  
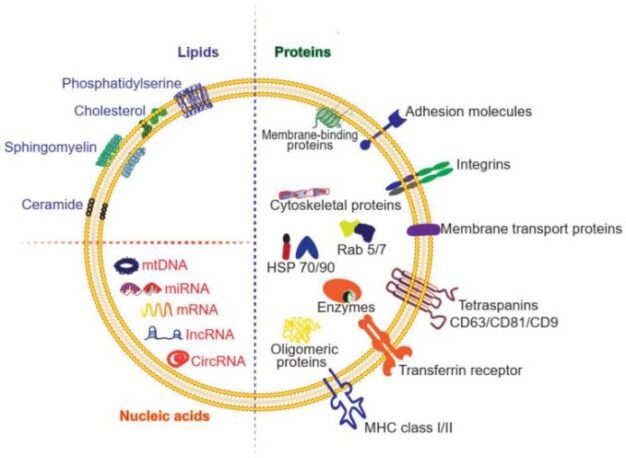
Figure 2: Composition of exosomes. Exosomes are composed of proteins (membrane-bound and soluble), lipids, and nucleic acids.
Credit: Liang Y, Duan L et.al., doi: 10.7150/thno.52570
Reproduced under the Creative Commons licenseÂ
Advantages of Native EVs:
Diverse Cargo:
Native EVs offer several features that make them promising for therapy and drug delivery. They can carry and protect a wide array of cargos, interact with target cells, and transfer bioactive molecules to these cells. The EV surface is adorned with molecules such as tetraspanins, integrins, and cell-specific proteins, which serve as natural targeting agents. These molecules enhance intercellular communication, ensuring EVs reach target tissues, bind to specific cells, and deliver their cargo.Â
Biocompatibility:
EVs also exhibit exceptional biocompatibility due to their origin from host cells, significantly reducing the risk of immunogenic responses that challenge traditional drug delivery systems. They are less immunogenic than parent cells, likely due to fewer transmembrane proteins like MHC complexes. EVs are more stable and have a longer shelf life than live cells, allowing for extended storage and transport. Unlike live cells, EVs do not risk uncontrolled growth or differentiation, minimising the chance of tumour formation. Additionally, EVs possess an intrinsic ability to cross biological barriers, including cellular and tissue barriers.Â
Limitations of Native EVs:
Uncontrolled Composition:
The natural cargo of native EVs is inherently heterogeneous, influenced by the cell of origin and its physiological state. Therefore, this limits the large-scale production of native EVs with similar biological functions. Native EVs present a challenge in terms of standardisation due to their inherently heterogeneous cargo. This variability arises from two main factors – (i) Cell of Origin -The specific cell type that releases the EV significantly influences its cargo composition. EVs from different cell types will naturally carry different sets of proteins, lipids, and nucleic acids, reflecting their unique functions.; (ii) Physiological State – The physiological state of the parent cell also plays a crucial role. For example, EVs released by a stressed or diseased cell may contain a distinct cargo profile compared to those from a healthy cell. This inherent variability in cargo composition presents a hurdle for large-scale production of native EVs with consistent and predictable biological effects. Â
Targeting Challenges:
While native EVs possess surface proteins that can facilitate some level of targeting, these mechanisms primarily promote local communication with neighbouring cells. This approach proves beneficial for influencing cells within close proximity, but it falls short when aiming for specific organs or tissues. The surface proteins on native EVs often lack the necessary specificity to bind exclusively to desired cell types. This can lead to off-target effects, where EVs deliver their cargo to unintended cells, potentially causing unwanted side effects. In some cases, EVs exhibit a tendency to return to their cell of origin. For example, EVs released from cancer cells may preferentially bind back to those same cancer cells, hindering their therapeutic application. When administered systemically, native EVs often accumulate non-specifically in organs like the liver and spleen. This indicates a lack of precise targeting mechanisms, limiting the delivery of the therapeutic cargo to the desired site of action. These limitations in targeting necessitate the development of engineered EVs, which can be modified to overcome these challenges and deliver their cargo with greater precision and efficacy.Â
Engineered EVs: Supercharged for Specificity
Engineered EVs represent a revolutionary advancement in the field of extracellular vesicle research. By addressing limitations present in native EVs, engineers are unlocking their full potential as therapeutic tools. Here’s how engineered EVs are transforming medicine:
Advantages of Engineered EVs:
Improved Targeting:
One of the most significant advantages of engineered EVs lies in their ability to achieve superior targeting. Unlike their native counterparts, engineered EVs can be customised to reach specific organs, tissues, or even individual cell types. This remarkable feat is achieved by attaching targeting molecules like antibodies or peptides to the EV surface. These molecules act like a postal code, specifically recognising and binding to receptors found only on the desired cells. This targeted binding significantly improves the accuracy of delivery, ensuring the therapeutic cargo reaches its intended recipient with minimal off-target effects on healthy tissues.Â
For example, researchers successfully engineered EVs with an amino acid peptide (GE11) that specifically binds to EGFR, a protein found on cancer cells. In a study, these modified exosomes were used to deliver microRNA (miRNA) to EGFR-expressing cancer tissues, indicating the potential to use this approach to revolutionise miRNA replacement therapies for various cancers.
Increase Circulation time:
Naturally occurring EVs have a limited lifespan in the bloodstream. Engineered EVs can be modified to prolong their circulation time, allowing them to reach their target more effectively. One strategy involves coating the EVs with polyethylene glycol (PEG). It was found that PEGylation can significantly increase circulation time from a mere 10 minutes (unmodified EVs) to a full hour. Another approach involves displaying CD47 on the EV surface. This molecule protects EVs from being engulfed by immune cells called monocytes and macrophages, leading to increased retention in the bloodstream. Additionally, EVs can be decorated with albumin, by binding to the albumin binding domains engineered into the extracellular loops of tetraspanins, to further extend their circulation time. Â
Tailored Cargo Loading:Â
Native EVs carry a limited amount of therapeutic cargo. Engineered EVs address this limitation by allowing scientists to significantly enhance the loading of drugs, proteins, or RNA molecules. This translates to more potent therapeutic effects. Engineered EVs offer the ability to be loaded with specific therapeutic cargo, such as drugs, genes, or silencing RNA. This empowers a more targeted treatment approach, delivering the desired molecules directly to diseased cells while minimising off-target effects. Examples of such therapeutic cargo include BCR-ABL siRNA or Imatinib, which can inhibit cancer cell growth in chronic myelogenous leukemia.Â
Limitations of Engineered EVs:
Limited Targeting and Uptake:
Current modification methods don’t significantly alter the natural targeting mechanisms of EVs. This means engineered EVs might still mimic the biodistribution and uptake patterns of native EVs, limiting their ability to reach specific target cells within the body.Â
Off-target Effects:
While targeting strategies are under development, engineered EVs can still interact with unintended cells. A deeper understanding of how these modified EVs interact with specific tissues is crucial to minimise off-target effects and improve tissue-specific targeting.Â
Unforeseen Side Effects:
The natural cargo present in EVs, alongside the therapeutic cargo loaded during engineering, can potentially lead to unexpected side effects. Researchers need to precisely analyse not only the EV content but also its interaction with target tissues and potential impacts on their function.Â
Production Bottlenecks:
Clinical translation of engineered EVs faces hurdles related to production. Cells naturally secrete a limited number of EVs, making large-scale production difficult. Additionally, current isolation methods are either slow or expensive. Standardised protocols for scalable production with consistent properties and quality control are still lacking.Â
Conclusion
Despite these limitations, engineered EVs represent a significant leap forward in developing targeted therapies. Their ability to combine the natural benefits of EVs with precise targeting and cargo delivery offers immense potential. From selectively attacking cancer cells to treating genetic disorders, engineered EVs hold promise for personalised medicine with minimal side effects. As research progresses and overcomes these challenges, engineered EVs have the potential to revolutionize how we treat a wide range of diseases.
References
Belhadj Z, He B, Deng H, Song S, Zhang H, Wang X, Dai W, Zhang Q. A combined “eat me/don’t eat me” strategy based on extracellular vesicles for anticancer nanomedicine. J Extracell Vesicles. 2020 Aug 19;9(1):1806444. doi: 10.1080/20013078.2020.1806444.
Bellavia D, Raimondo S, Calabrese G, et al. Interleukin 3- receptor targeted exosomes inhibit in vitro and in vivo Chronic Myelogenous Leukemia cell growth. Theranostics. 2017;7(5):1333-1345. Published 2017 Mar 16. doi:10.7150/thno.17092
Bobis-Wozowicz S, Marbán E. Editorial: Extracellular Vesicles as Next Generation Therapeutics. Front Cell Dev Biol. 2022 May 12;10:919426. doi: 10.3389/fcell.2022.919426.
Elsharkasy OM, Nordin JZ, Hagey DW, et al. Extracellular vesicles as drug delivery systems: Why and how?. Adv Drug Deliv Rev. 2020;159:332-343. doi:10.1016/j.addr.2020.04.004
Frolova L, Li ITS. Targeting Capabilities of Native and Bioengineered Extracellular Vesicles for Drug Delivery. Bioengineering (Basel). 2022;9(10):496. Published 2022 Sep 22. doi:10.3390/bioengineering9100496
Kooijmans SAA, Fliervoet LAL, van der Meel R, et al. PEGylated and targeted extracellular vesicles display enhanced cell specificity and circulation time. J Control Release. 2016;224:77-85. doi:10.1016/j.jconrel.2016.01.009
Liang X, Niu Z, Galli V, et al. Extracellular vesicles engineered to bind albumin demonstrate extended circulation time and lymph node accumulation in mouse models. J Extracell Vesicles. 2022;11(7):e12248. doi:10.1002/jev2.12248
Liang Y, Duan L, Lu J, Xia J. Engineering exosomes for targeted drug delivery. Theranostics. 2021 Jan 1;11(7):3183-3195. doi: 10.7150/thno.52570.
Murphy DE, de Jong OG, Brouwer M, Wood MJ, Lavieu G, Schiffelers RM, Vader P. Extracellular vesicle-based therapeutics: natural versus engineered targeting and trafficking. Exp Mol Med. 2019 Mar 15;51(3):1-12. doi: 10.1038/s12276-019-0223-5.
Ohno S, Takanashi M, Sudo K, Ueda S, Ishikawa A, Matsuyama N, Fujita K, Mizutani T, Ohgi T, Ochiya T, Gotoh N, Kuroda M. Systemically injected exosomes targeted to EGFR deliver antitumor microRNA to breast cancer cells. Mol Ther. 2013 Jan;21(1):185-91. doi: 10.1038/mt.2012.180.
Qiao L, Hu S, Huang K, Su T, Li Z, Vandergriff A, Cores J, Dinh PU, Allen T, Shen D, Liang H, Li Y, Cheng K. Tumor cell-derived exosomes home to their cells of origin and can be used as Trojan horses to deliver cancer drugs. Theranostics. 2020 Feb 10;10(8):3474-3487. doi: 10.7150/thno.39434.
Sun Y, Sun F, Xu W, Qian H. Engineered Extracellular Vesicles as a Targeted Delivery Platform for Precision Therapy. Tissue Eng Regen Med. 2023 Apr;20(2):157-175. doi: 10.1007/s13770-022-00503-y.
Smyth T, Kullberg M, Malik N, Smith-Jones P, Graner MW, Anchordoquy TJ. Biodistribution and delivery efficiency of unmodified tumor-derived exosomes. J Control Release. 2015 Feb 10;199:145-55. doi: 10.1016/j.jconrel.2014.12.013.