Summary
Get an insightful overview of the burgeoning field of cell and gene therapies, showcasing their potential to revolutionize medical treatments. Discover the power of cell therapy, from its diverse cell sources to its remarkable efficacy in treating diseases. Then, delve into the realm of gene therapy, where we’ll unveil the intricate process of editing the genetic blueprint to combat disorders. Through real-life stories, witness the transformative potential of gene therapy and its promise in curing genetic diseases. Gain insight into the intertwined nature of cell and gene therapies and explore the latest progress in research and clinical implementation with a comprehensive list of FDA-approved therapies as of 2024. Don’t miss this chance to stay informed and intrigued by the dynamic landscape of innovation in healthcare. Dive into the article now and unlock the future of medicine!
Cell and gene therapy are both rapidly advancing areas of modern medicine, representing groundbreaking approaches that hold immense promise for revolutionising the way we treat diseases. But with all the buzz, it can be easy to get confused about what sets them apart.
In this blog post, we’ll break down the key differences between cell and gene therapy, giving you a clearer picture of these innovative approaches.
Cell Therapy: Introducing the Cavalry
Cell therapy uses healthy cells to treat a variety of diseases. It uses healthy cells, to replace diseased or damaged ones.
Typically, it involves taking healthy cells from the patient’s own body (autologous) or a donor (allogeneic), and the cells are then grown and expanded in a lab setting. Sometimes, the cells might be genetically modified to make them even more effective at fighting disease. Finally, the healthy cells are infused back into your body through an intravenous (IV) or injection.
Sources of the Cells Used for Cell Therapy:
- Autologous (auto = self): Here, the cells used for therapy come from the patient themself. Doctors extract healthy cells from the patient’s body, expand them in a lab, and then reintroduce them to fight the disease. This approach minimises the risk of rejection as the body is receiving its own cells.
- Allogeneic (allo = other): In this case, the cells for therapy come from a donor, either a matched sibling or an unrelated donor from a registry. Matching the donor’s and recipient’s human leukocyte antigens (HLA) is crucial to minimise rejection. Allogeneic cells can offer some advantages, like having a potentially stronger anti-tumour effect in some cancers.
Table 1: Differences Between Autologous and Allogeneic Cell Therapy
Feature | Autologous Cell Therapy | Allogeneic Cell Therapy |
Cell Source | Patient’s own body | Donor (matched sibling or unrelated) |
Risk of Rejection | Lower | Higher, requires careful HLA matching |
Availability of Cells | May be limited depending on the patient’s health | More readily available |
Manufacturing Time | Longer, as cells need to be expanded from the patient | Shorter, as cells may be readily available from a donor registry |
Examples of Cell Therapy:
- Bone Marrow Transplants: This is a well-established cell therapy. They’ve been around for decades and have saved countless lives. It involves harvesting the hematopoietic progenitor cells (HPCs) from the bone marrow or the umbilical cord blood. These cells are versatile, and can turn into any type of blood cell the body needs. Treatments using these cells aim to establish healthy blood cell production in individuals whose blood cells or immune cells are not working properly. It is used to treat various blood cancers and other blood disorders.
- Chimeric Antigen Receptor (CAR) T-cell Therapy: This is a newer and more targeted form of cell therapy used to treat some cancers. It involves taking a patient’s T cells, modifying them genetically in a lab, and reintroducing them into the patient’s body. Once infused back into the patient, the CAR T cells recognise and attack the cancer cells. This targeted approach offers a powerful way to eliminate cancer cells.
- Mesenchymal Stem Cells (MSCs) Therapy : MSCs are adult stem cells found in various tissues throughout the body, like bone marrow, adipose tissue (fat), and umbilical cord blood. These cells are versatile and MSCs be harvested for research or to be used as therapy to help the body heal in different ways depending on what is being targeted. MSC therapy is still under development, but it’s being explored for a variety of conditions, including autoimmune diseases, heart diseases, musculoskeletal disorders, and Graft-versus-host disease (GVHD).
Gene Therapy: Editing the Blueprint
Gene therapy is a technique that uses genetic material to treat or prevent disease. The genetic material that is delivered works by replacing the faulty gene with a healthy one, inactivating a harmful gene, or adding a new or modified gene to help the body fight disease. The genetic material is delivered into the cells by delivery vehicles called the vectors.
Types of Vectors Used in Gene Therapy:
Gene therapy relies on tiny carriers called vectors to deliver therapeutic genes into target cells. These vectors come in two main categories, each with its own advantages and limitations:
- Viral Vectors: These commonly used vectors are derived from viruses like adenoviruses, adeno-associated viruses (AAVs), retroviruses, or lentiviruses. To ensure safety, scientists remove all the viral genes from these vectors and modify them to carry only the therapeutic gene. Since viruses have natural mechanisms for entering cells, viral vectors are efficient delivery vehicles. However, there are potential safety concerns, such as triggering immune responses or causing insertional mutagenesis (unintended insertion of the gene into the host’s genome).
- Non-viral Vectors: These are alternative vectors that don’t use viruses. Examples include plasmids (circular DNA molecules), liposomes, polymers, or nanoparticles. They generally have a lower risk of insertional mutagenesis, but their efficiency in delivering the gene to target cells can be lower compared to viral vectors.
Delivering the Vectors: Targeting the Right Cells
The delivery method for gene therapy vectors depends on two key factors: the type of vector being used and the target cells it needs to reach. Here are the two main approaches:
- In Vivo Delivery: This is the most common method, especially for viral vectors. The vector solution is directly injected into the patient, targeting the specific tissue, bloodstream, or body cavity relevant to the disease.
- For example, for liver diseases, the vector might be injected directly into the liver.
- Ex Vivo Delivery: This approach is used for specific cell types, often blood cells. A patient’s cells are extracted, treated with the vector in a controlled laboratory setting (ex vivo), and then reintroduced into the patient’s body.
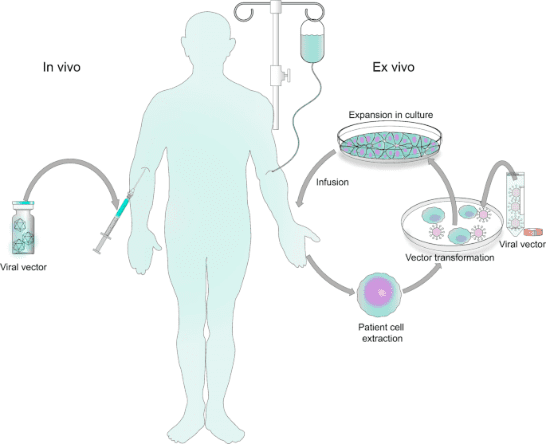
Figure 1: In Vivo and Ex Vivo delivery of viral vectors.
Credit: Bulcha, J.T., Wang, Y., Ma, H. et al. https://doi.org/10.1038/s41392-021-00487-6
Reproduced under the Creative Commons license
Choosing the Right Delivery Method:
The choice between in vivo and ex vivo delivery depends on several factors:
- Target cells: Some vectors are better suited for specific cell types. Ex vivo delivery might be necessary if the target cells are difficult to reach directly within the body.
- Vector characteristics: Viral vectors often favour in vivo delivery due to their natural ability to enter cells. Non-viral vectors might require ex vivo treatment for improved delivery efficiency.
- Disease characteristics: The location and accessibility of the target tissue also influence the choice of delivery method.
Correcting the Blueprint of Life: Examples of Gene Therapy in Action
Gene therapy offers a revolutionary approach to treating diseases by targeting the root cause – faulty genes. This is particularly promising for rare diseases, where most cases are caused by a single identifiable genetic mutation. Unlike medications that address symptoms, gene therapy has the potential to offer a permanent cure.
Several groundbreaking advancements in gene therapy have emerged in recent years:
- Restoring Vision: Leber congenital amaurosis (LCA) is a group of inherited retinal diseases that can lead to blindness. In 2017, Luxturna became the first FDA-approved gene therapy for LCA. This therapy works by delivering a healthy copy of the mutated gene directly to retinal cells, potentially restoring vision function.
- Treating Blood Disorders: Gene therapy is also showing promise in treating blood diseases caused by single-gene mutations. Examples include:
- Sickle cell disease: This condition causes the production of abnormal red blood cells. Gene therapy approaches aim to introduce a healthy version of the mutated gene, enabling production of normal red blood cells.
- Beta thalassemia: This disease reduces hemoglobin production. Gene therapy is being explored to deliver a functional copy of the missing gene responsible for hemoglobin production.
- Addressing Hemophilia: Hemophilia patients lack specific clotting factors, leading to excessive bleeding. Two FDA-approved gene therapies utilizing adeno-associated virus (AAV) vectors have been developed for Hemophilia A and B. These therapies aim to introduce genes that instruct cells to produce the missing clotting factors.
- Combating Spinal Muscular Atrophy (SMA): SMA is a neurodegenerative disease affecting motor neurons. In 2019, Zolgensma became the first gene therapy approved for treating children under two years old with SMA. This therapy replaces the faulty gene responsible for the disease, potentially halting or reversing its progression.
The Blurring Lines and Future Potential
While distinct, cell and gene therapy can sometimes overlap. CAR T-cell therapy, for instance, exemplifies the overlap between these fields. This treatment for certain cancers involves genetically modifying a patient’s immune cells (T cells) to recognise and attack cancer cells.
Both gene therapy and cell therapy are rapidly evolving fields with immense potential. Researchers are constantly working on improving their safety, effectiveness, and reach, aiming to tackle a wider range of conditions, including complex diseases like cancer. The future of medicine looks bright with these innovative therapeutic approaches.
Current FDA Approved Cell & Gene Therapies
To date in 2024, the U.S. Food and Drug Administration (FDA) has approved two new cell and gene therapies, bringing the total number of approved therapies to 36. These encompass a variety of approaches targeting different diseases. This is a significant number and reflects the rapid advancements in this field. The table below contains all licensed products listed as ‘Approved Cellular and Gene Therapy Products’ on fda.gov (last updated on 18/03/2024).
Table 2: List of FDA-approved cell and gene therapies.
Name | Therapeutics | Manufacturer | Indications |
CAR T Cells-based Therapy | |||
KYMRIAH (2017) | Autologous CAR T cells expressing anti-CD19 CAR transgene via lentiviral vector | Novartis Pharmaceuticals Corporation | For adult patients with certain kinds of relapsed or refractory follicular lymphoma |
YESCARTA (2017) | Autologous CAR T cells expressing anti-CD19 CAR transgene via retroviral vector | Kite Pharma, Inc. | For adult patients with certain kinds of relapsed or refractory large B-cell lymphoma |
TECARTUS (2020) | Autologous CAR T cells expressing anti-CD19 CAR transgene via retroviral vector | Kite Pharma, Inc. | For adult patients with certain kinds of relapsed or refractory mantle cell lymphoma |
ABECMA (2021) | Autologous CAR T cells expressing anti-B-cell maturation antigen (BCMA) CAR transgene via lentiviral vector | Celgene Corporation, a Bristol-Myers Squibb Company | For adult patients with certain kinds of relapsed or refractory multiple myeloma |
BREYANZI (2021) | Autologous CAR T cells expressing anti-CD19 CAR transgene via lentiviral vector | Juno Therapeutics, Inc., a Bristol-Myers Squibb Company | For adult patients with certain kinds of large B-cell lymphoma |
CARVYKTI (2022) | Autologous CAR T Cells expressing anti-BCMA CAR transgene via lentiviral vector | Janssen Biotech, Inc. | For adult patients with certain kinds of relapsed or refractory multiple myeloma |
Immune Cells-based Therapy | |||
PROVENGE (2010) | Autologous antigen-presenting cells (APC) activated with prostatic acid phosphatase (PAP)- granulocyte-macrophage colony-stimulating factor (GM-CSF), PAP-GM-CSF | Dendreon Corp. | For treatment of certain types of prostate cancer |
AMTAGVI (2024) | Autologous tumour-derived T cells | Iovance Biotherapeutics, Inc. | For adult patients with unresectable or metastatic melanoma previously treated with a PD-1 blocking antibody, and if BRAF V600 mutation positive, a BRAF inhibitor with or without a MEK inhibitor |
Hematopoietic Progenitor Cell-based Therapy | |||
HEMACORD (2011) | Allogenic hematopoietic progenitor cells; Cord blood | New York Blood Center | For hematopoietic and immunologic reconstitution in patients with disorders affecting the hematopoietic system |
DUCORD (2012) | Allogenic hematopoietic progenitor cells; Cord blood | Duke University School of Medicine | For hematopoietic and immunologic reconstitution in patients with disorders affecting the hematopoietic system |
HPC, Cord Blood (2012) | Allogenic hematopoietic progenitor cells; Cord blood | Clinimmune Labs, University of Colorado Cord Blood Bank | For hematopoietic and immunologic reconstitution in patients with disorders affecting the hematopoietic system |
ALLOCORD (2013) | Allogenic hematopoietic progenitor cells; Cord blood | SSM Cardinal Glennon Children’s Medical Center | For hematopoietic and immunologic reconstitution in patients with disorders affecting the hematopoietic system |
CLEVECORD (2016) | Allogenic hematopoietic progenitor cells; Cord blood | Cleveland Cord Blood Center | For hematopoietic and immunologic reconstitution in patients with disorders affecting the hematopoietic system |
HPC, Cord Blood (2016) | Allogenic hematopoietic progenitor cells; Cord blood | LifeSouth Community Blood Centers, Inc. | For hematopoietic and immunologic reconstitution in patients with disorders affecting the hematopoietic system |
HPC, Cord Blood (2016) | Allogenic hematopoietic progenitor cells; Cord blood | Bloodworks | For hematopoietic and immunologic reconstitution in patients with disorders affecting the hematopoietic system |
HPC, Cord Blood (2018) | Allogenic hematopoietic progenitor cells; Cord blood | MD Anderson Cord Blood Bank | For hematopoietic and immunologic reconstitution in patients with disorders affecting the hematopoietic system |
OMISIRGE (2023) | Nicotinamide modified allogenic hematopoietic progenitor cell | Gamida Cell Ltd. | For use in adults and pediatric patients 12 years and older with hematologic malignancies |
Other Cell-based Therapy | |||
LAVIV (2011) | Autologous dermal fibroblasts | Fibrocell Technologies | For improvement in the appearance of moderate to severe nasolabial fold wrinkles in adults |
GINTUIT (2012) | Cellularised scaffold of allogeneic keratinocytes and dermal fibroblasts, human extracellular matrix proteins, and bovine collagen | Organogenesis Inc. | For topical application to a surgically created vascular wound bed in the treatment of mucogingival conditions in adults |
MACI (2016) | Cellularised scaffold of autologous chondrocytes on porcine collagen membrane | Vericel Corp. | For repair of cartilage defects of the knee in adults |
STRATAGRAFT (2021) | Cellularised scaffold of allogenic keratinocytes and dermal fibroblasts in murine collagen | Stratatech Corporation | For adult patients with some types of thermal burns |
RETHYMIC (2021) | Allogenic thymus tissue | Enzyvant Therapeutics GmbH | For immune reconstitution in pediatric patients with congenital athymia |
LANTIDRA (2023) | Allogenic pancreatic islet cells from deceased donor | CellTrans Inc. | For adult patients with certain types of type I diabetes |
CD34+ HSC-based Gene Therapy | |||
SKYSONA (2022) | Autologous CD34+ hematopoietic stem cells (HSC) engineered with Lenti-D vectors expressing the ABCD1 gene | bluebird bio, Inc. | For patients with a kind of cerebral adrenoleukodystrophy |
ZYNTEGLO (2022) | Autologous CD34+ HSC engineered with a BB305 lentivirus encoding β-globin gene (βA-T87Q-globin gene) | bluebird bio, Inc. | For adult and pediatric patients with certain types of ß-thalassemia |
CASGEVY (2023) | Autologous CD34+ HSC engineered with electroporation of CRISPR/Cas9 RNP complexes to decrease expression of BCL11A to increase foetal hemoglobin production | Vertex Pharmaceuticals, Inc. | For patients 12 years and older with certain kinds of sickle cell disease |
LYFGENIA (2023) | Autologous CD34+ HSC engineered with a BB305 lentivirus encoding β-globin gene (βA-T87Q-globin gene) | bluebird bio, Inc. | For patients 12 years of age or older with certain kinds of sickle cell disease |
LENMELDY (2024) | Autologous CD34+ HSC engineered with a lentivirus encodingthe human arylsulfatase A (ARSA) gene | Orchard Therapeutics (Europe) Limited | For children with certain kinds of metachromatic leukodystrophy (MLD) |
Adenoviral/Adeno-associated viral-based Gene Therapy | |||
LUXTURNA (2017) | A recombinant adeno-associated virus (AAV2) containing a transgene encoding RPE65 | Spark Therapeutics, Inc. | For patients with RPE65-mutation-associated retinal dystrophy |
ZOLGENSMA (2019) | A recombinant adeno-associated virus (AAV9) containing a transgene encoding the human survival motor neuron (SMN) protein | Novartis Gene Therapies, Inc. | For pediatric patients with certain types of spinal muscular atrophy |
ADSTILADRIN (2022) | A recombinant adenovirus containing a transgene encoding the human interferon alfa-2b (IFNα2b) | Ferring Pharmaceuticals A/S | For adult patients with certain types of bladder cancer |
HEMGENIX (2022) | A recombinant adeno-associated virus (AAV) containing a transgene encoding the gain-of-function Padua variant of human Factor IX (variant R338L) | CSL Behring LLC | For adult patients with certain kinds of Hemophilia B |
ELEVIDYS (2023) | A recombinant adeno-associated virus (AAVrh74) containing a transgene encoding the micro-dystrophin protein | Sarepta Therapeutics, Inc. | For pediatric patients with certain types of Duchenne muscular dystrophy |
ROCTAVIAN (2023) | A recombinant adeno-associated virus (AAV5) containing a transgene encoding the B-domain deleted SQ form of the human coagulation factor VIII (hFVIII-SQ) | BioMarin Pharmaceutical Inc. | For adult patients with certain types of hemophilia A |
Oncolytic Viral-based Gene Therapy | |||
IMLYGIC (2015) | Recombinant HSV-1 expressing huGM-CSF to promote an anti-tumour immune response | BioVex, Inc., a subsidiary of Amgen Inc. | Local treatment in patients with certain unresectable melanoma lesions |
VYJUVEK (2023) | Recombinant HSV-1expressing collagen type VII gene (COL7) | Krystal Biotech, Inc. | Topical treatment of certain wounds in patients with dystrophic epidermolysis bullosa with mutations in the COL7A1 gene |
References:
Bashor, C.J., Hilton, I.B., Bandukwala, H. et al. Engineering the next generation of cell-based therapeutics. Nat Rev Drug Discov 21, 655–675 (2022). https://doi.org/10.1038/s41573-022-00476-6
Bulcha, J.T., Wang, Y., Ma, H. et al. Viral vector platforms within the gene therapy landscape. Sig Transduct Target Ther 6, 53 (2021). https://doi.org/10.1038/s41392-021-00487-6
Papaioannou I, Owen JS, Yáñez-Muñoz RJ. Clinical applications of gene therapy for rare diseases: A review. Int J Exp Pathol. 2023 Aug;104(4):154-176. doi: 10.1111/iep.12478.
Wang LL, Janes ME, Kumbhojkar N, et al. Cell therapies in the clinic. Bioeng Transl Med. 2021;6(2):e10214. Published 2021 Feb 26. doi:10.1002/btm2.10214